User login
Febrile Infant Diagnosis Code Accuracy
Fever is one of the most common reasons for emergency department (ED) evaluation of infants under 90 days of age.[1] Up to 10% to 20% of febrile young infants will have a serious bacterial infection (SBI),[2, 3, 4] but infants with SBI are difficult to distinguish from those without SBI based upon symptoms and physical examination findings alone.[5] Previously developed clinical prediction algorithms can help to identify febrile infants at low risk for SBI, but differ in age range as well as recommendations for testing and empiric treatment.[6, 7, 8] Consequently, there is widespread variation in management of febrile young infants at US children's hospitals,[9, 10, 11] and defining optimal management strategies remains an important issue in pediatric healthcare.[12] Administrative datasets are convenient and inexpensive, and can be used to evaluate practice variation, trends, and outcomes of a large, diverse group of patients within and across institutions.[9, 10] Accurately identifying febrile infants evaluated for suspected SBI in administrative databases would facilitate comparative effectiveness research, quality improvement initiatives, and institutional benchmarking.
Prior studies have validated the accuracy of administrative billing codes for identification of other common childhood illnesses, including urinary tract infection (UTI)[13] and pneumonia.[14] The accuracy of International Classification of Diseases, Ninth Revision (ICD‐9) diagnosis codes in identifying febrile young infants evaluated for SBI is not known. Reliance on administrative ICD‐9 diagnosis codes for patient identification can lead to misclassification of patients due to variable database quality, the validity of the diagnosis codes being utilized, and hospital coding practices.[15] Additionally, fever is a symptom and not a specific diagnosis. If a particular bacterial or viral diagnosis is established (eg, enterovirus meningitis), a discharge diagnosis of fever may not be attributed to the patient encounter. Thus, evaluating the performance characteristics and capture of clinical outcomes of different combinations of ICD‐9 diagnosis codes for identifying febrile infants is necessary for both the conduct and interpretation of studies that utilize administrative databases. The primary objective of this investigation was to identify the most accurate ICD‐9 coding strategies for the identification of febrile infants aged <90 days using administrative data. We also sought to evaluate capture of clinically important outcomes across identification strategies.
METHODS
Study Design and Setting
For this multicenter retrospective study, we used the Pediatric Health Information System (PHIS) database to identify infants <90 days of age[16] who presented between July 1, 2012 and June 30, 2013 to 1 of 8 EDs. We assessed performance characteristics of ICD‐9 diagnosis code case‐identification algorithms by comparing ICD‐9 code combinations to a fever reference standard determined by medical record review. The institutional review board at each participating site approved the study protocol.
Data Source
Data were obtained from 2 sources: the PHIS database and medical record review. We used the PHIS database to identify eligible patients by ICD‐9 diagnosis codes; patient encounters were randomly selected using a random number generator. The PHIS database contains demographic, diagnosis, and billing data from 44 hospitals affiliated with the Children's Hospital Association (Overland Park, Kansas) and represents 85% of freestanding children's hospitals in the United States.[17] Data are deidentified; encrypted unique patient identifiers permit tracking of patients across visits within a site.[18] The Children's Hospital Association and participating hospitals jointly assure the quality and integrity of the data.[19]
For each patient encounter identified in the PHIS database, detailed medical record review was performed by trained investigators at each of the 8 study sites (see Supporting Information, Appendix, in the online version of this article). A standardized data collection instrument was pilot tested by all investigators prior to use. Data were collected and managed using the Research Electronic Data Capture (REDCap) tool hosted at Boston Children's Hospital.[20]
Exclusions
Using PHIS data, prior to medical record review we excluded infants with a complex chronic condition as defined previously[21] and those transferred from another institution, as these infants may warrant a nonstandard evaluation and/or may have incomplete data.
ICD‐9 Diagnosis Code Groups
In the PHIS database, all patients discharged from the hospital (including hospitalized patients as well as patients discharged from the ED) receive 1 or more ICD‐9 discharge diagnosis codes. These diagnosis codes are ascribed after discharge from the hospital, or for ED patients, after ED discharge. Additionally, patients may receive an admission diagnosis, which reflects the diagnosis ascribed at the time of ED discharge or transfer to the inpatient unit.
We reviewed medical records of infants selected from the following ICD‐9 diagnosis code groups (Figure 1): (1) discharge diagnosis code of fever (780.6 [fever and other physiologic disturbances of temperature regulation], 778.4 [other disturbances of temperature regulation of newborn], 780.60 [fever, unspecified], or 780.61 [fever presenting with conditions classified elsewhere])[9, 10] regardless of the presence of admission diagnosis of fever or diagnosis of serious infection, (2) admission diagnosis code of fever without associated discharge diagnosis code of fever,[10] (3) discharge diagnosis code of serious infection determined a priori (see Supporting Information, Appendix, in the online version of this article) without discharge or admission diagnosis code of fever, and (4) infants without any diagnosis code of fever or serious infection.
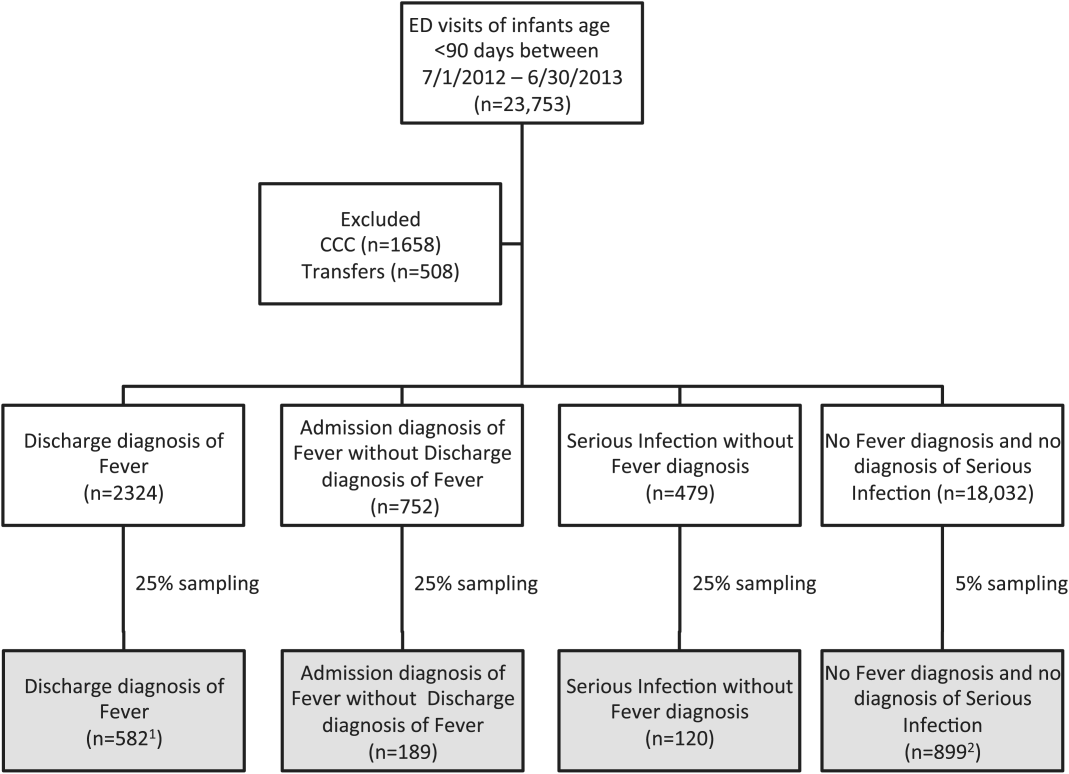
Medical records reviewed in each of the 4 ICD‐9 diagnosis code groups were randomly selected from the overall set of ED encounters in the population of infants <90 days of age evaluated during the study period. Twenty‐five percent population sampling was used for 3 of the ICD‐9 diagnosis code groups, whereas 5% sampling was used for the no fever/no serious infection code group. The number of medical records reviewed in each ICD‐9 diagnosis code group was proportional to the distribution of ICD‐9 codes across the entire population of infants <90 days of age. These records were distributed equally across sites (228 records per site), except for 1 site that does not assign admission diagnoses (201 records).
Investigators were blinded to ICD‐9 diagnosis code groups during medical record review. Infants with multiple visits during the study period were eligible to be included more than once if the visits occurred more than 3 days apart. For infants with more than 1 ED visit on a particular calendar day, investigators were instructed to review the initial visit.
For each encounter, we also abstracted demographic characteristics (gender, race/ethnicity), insurance status, hospital region (using US Census categories[22]), and season from the PHIS database.
Reference Standard
The presence of fever was determined by medical record review. We defined fever as any documented temperature 100.4F (38.0C) at home or in the ED.[16]
ICD‐9 Code Case‐Identification Algorithms
Using the aforementioned ICD‐9 diagnosis code groups individually and in combination, the following 4 case‐identification algorithms, determined from prior study or group consensus, were compared to the reference standard: (1) ICD‐9 discharge diagnosis code of fever,[9] (2) ICD‐9 admission or discharge diagnosis code of fever,[10, 11] (3) ICD‐9 discharge diagnosis code of fever or serious infection, and (4) ICD‐9 discharge or admission diagnosis code of fever or serious infection. Algorithms were compared overall, separately for discharged and hospitalized infants, and across 3 distinct age groups (28 days, 2956 days, and 5789 days).
Patient‐Level Outcomes
To compare differences in outcomes by case‐identification algorithm, from the PHIS database we abstracted hospitalization rates, rates of UTI/pyelonephritis,[13] bacteremia/sepsis, and bacterial meningitis.[19] Severe outcomes were defined as intensive care unit admission, mechanical ventilation, central line placement, receipt of extracorporeal membrane oxygenation, or death. We assessed hospital length of stay for admitted infants and 3‐day revisits,[23, 24] and revisits resulting in hospitalization for infants discharged from the ED at the index visit. Patients billed for observation care were classified as being hospitalized.[25, 26]
Data Analysis
Accuracy of the 4 case‐identification algorithms (compared with the reference standard) was calculated using sensitivity, specificity, negative predictive value (NPV), and positive predictive value (PPV), along with 95% confidence interval (CI). Prior to analysis, a 5‐fold weighting factor was applied to the no fever/no serious infection group to account for the differential sampling used for this group (5% vs 25% for the other 3 ICD‐9 diagnosis code groups). This weighting was done to approximate the true prevalence of each ICD‐9 code group within the larger population, so that an accurate rate of false negatives (infants with fever who had neither a diagnosis of fever nor serious infection) could be calculated.
We described continuous variables using median and interquartile range or range values and categorical variables using frequencies with 95% CIs. We compared categorical variables using a 2 test. We determined statistical significance as a 2‐tailed P value <0.05. Statistical analyses were performed using SAS version 9.3 (SAS Institute, Cary, NC).
RESULTS
Study Patients
During the 1‐year study period, 23,753 ED encounters for infants <90 days of age were identified in the PHIS database at the 8 participating sites. Of these infant encounters, 2166 (9.2%) were excluded (1658 infants who had a complex chronic condition and 508 transferred into the ED), leaving 21,587 infants available for selection. After applying our sampling strategy, we identified 1797 encounters for medical record review. Seven encounters from 3 hospitals with missing medical records were excluded, resulting in a final cohort of 1790 encounters (Figure 1). Among included infants, 552 (30.8%) were 28 days, 743 (41.5%) were 29 to 56 days, and 495 (27.8%) were 57 to 89 days of age; 737 (41.2%) infants were hospitalized. Patients differed in age, race, payer, and season across ICD‐9 diagnosis code groups (see Supporting Information, Table 1, in the online version of this article).
ICD‐9 Diagnosis Code Algorithm | Overall | |||
---|---|---|---|---|
Sensitivity, % (95% CI) | Specificity, % (95% CI) | Negative Predictive Value, % (95% CI) | Positive Predictive Value, % (95% CI) | |
| ||||
Discharge diagnosis of fever | 53.2 (50.056.4) | 98.2 (97.898.6) | 90.8 (90.091.6) | 86.1 (83.388.9) |
Hospitalized | 47.3 (43.151.5) | 97.7 (96.998.5) | 80.6 (78.682.6) | 90.2 (86.893.6) |
Discharged from ED | 61.4 (56.666.2) | 98.4 (98.098.8) | 95.4 (94.796.1) | 82.1 (77.786.5) |
Discharge or admission diagnosis of Fever | 71.1 (68.274.0) | 97.7 (97.398.1) | 94.1 (93.494.8) | 86.9 (84.589.3) |
Hospitalized | 72.5 (68.876.2) | 97.1 (96.298.0) | 88.8 (87.190.5) | 91.7 (89.194.3) |
Discharged from ED | 69.2 (64.773.7) | 98.0 (97.598.5) | 96.3 (95.796.9) | 80.8 (76.685.0) |
Discharge diagnosis of fever or serious infection | 63.7 (60.666.8) | 96.5 (96.097.0) | 92.6 (91.893.4) | 79.6 (76.782.5) |
Hospitalized | 63.9 (59.967.9) | 92.5 (91.094.0) | 85.1 (83.287.0) | 79.1 (75.382.9) |
Discharged from ED | 63.4 (58.768.1) | 98.1 (97.698.6) | 95.6 (94.996.3) | 80.2 (75.884.6) |
Discharge or admission diagnosis of fever or serious infection | 76.6 (73.979.3) | 96.2 (95.696.8) | 95.1 (94.595.7) | 81.0 (78.483.6) |
Hospitalized | 80.8 (77.584.1) | 92.1 (90.693.6) | 91.5 (89.993.1) | 82.1 (78.985.3) |
Discharged from ED | 71.0 (66.575.5) | 97.7 (97.298.2) | 96.5 (95.997.1) | 79.4 (75.283.6) |
Among the 1790 patient encounters reviewed, a total of 766 infants (42.8%) met the reference standard definition for fever in the cohort. An additional 47 infants had abnormal temperature reported (documentation of tactile fever, history of fever without a specific temperature described, or hypothermia) but were classified as having no fever by the reference standard.
ICD‐9 Code Case‐Identification Algorithm Performance
Compared with the reference standard, the 4 case‐identification algorithms demonstrated specificity of 96.2% to 98.2% but lower sensitivity overall (Figure 2). Discharge diagnosis of fever alone demonstrated the lowest sensitivity. The algorithm of discharge or admission diagnosis of fever resulted in increased sensitivity and the highest PPV of all 4 algorithms (86.9%, 95% CI: 84.5‐89.3). Addition of serious infection codes to this algorithm resulted in a marginal increase in sensitivity and a similar decrease in PPV (Table 1). When limited to hospitalized infants, specificity was highest for the case‐identification algorithm of discharge diagnosis of fever and similarly high for discharge or admission diagnosis of fever; sensitivity was highest for the algorithm of discharge or admission diagnosis of fever or diagnosis of serious infection. For infants discharged from the ED, algorithm specificity was 97.7% to 98.4%, with lower sensitivity for all 4 algorithms (Table 1). Inclusion of the 47 infants with abnormal temperature as fever did not materially change algorithm performance (data not shown).
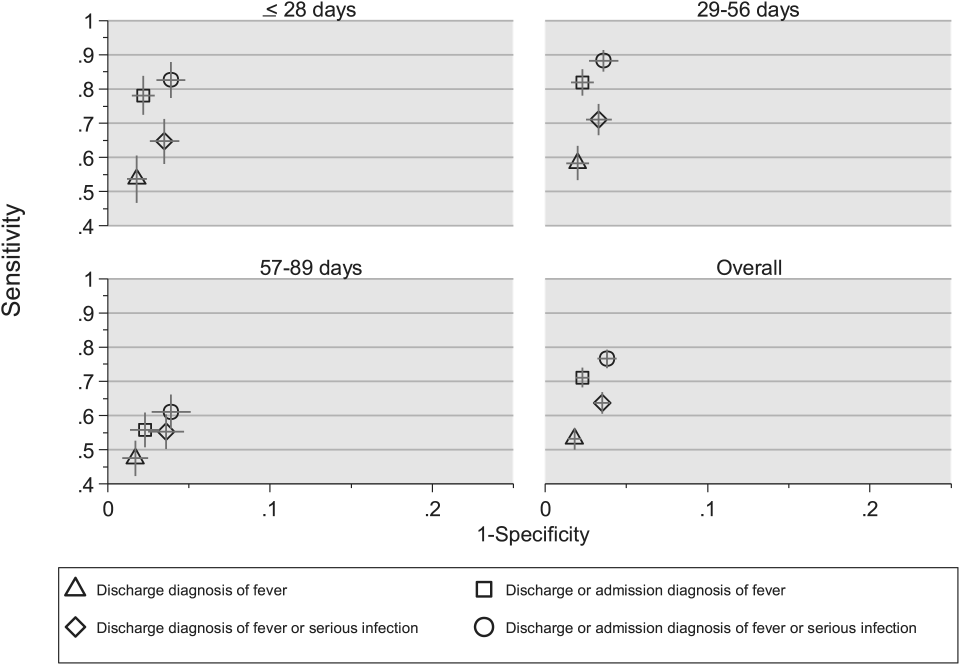
Across all 3 age groups (28 days, 2956 days, and 5789 days), the 4 case‐identification algorithms demonstrated specificity >96%, whereas algorithm sensitivity was highest in the 29‐ to 56‐days‐old age group and lowest among infants 57 to 89 days old across all 4 algorithms (Figure 2). Similar to the overall cohort, an algorithm of discharge or admission diagnosis of fever demonstrated specificity of nearly 98% in all age groups; addition of serious infection codes to this algorithm increased sensitivity, highest in the 29‐ to 56‐days‐old age group (Figure 2; see also Supporting Information, Table 2, in the online version of this article).
ICD‐9 Diagnosis Code Algorithm | Sensitivity, Median % (Range) | Specificity, Median % (Range) | Negative Predictive Value, Median % (Range) | Positive Predictive Value, Median % (Range) |
---|---|---|---|---|
| ||||
Discharge diagnosis of fever | 56.2 (34.681.0) | 98.3 (96.499.1) | 92.1 (83.297.4) | 87.7 (74.093.2) |
Discharge or Admission diagnosis of Fever | 76.7 (51.385.0) | 97.8 (96.298.7) | 95.6 (86.997.4) | 87.4 (80.092.9) |
Discharge diagnosis of fever or serious infection | 68.3 (44.287.3) | 96.5 (95.498.0) | 93.6 (85.298.2) | 78.3 (74.289.0) |
Discharge or admission diagnosis of fever or serious infection | 83.1 (58.390.7) | 95.8 (95.498.0) | 96.5 (88.598.2) | 79.1 (77.490.4) |
Across the 8 study sites, median specificity was 95.8% to 98.3% for the 4 algorithms, with little interhospital variability; however, algorithm sensitivity varied widely by site. Median PPV was highest for discharge diagnosis of fever alone at 87.7% but ranged from 74.0% to 93.2% across sites. Median PPV for an algorithm of discharge or admission diagnosis of fever was similar (87.4%) but with less variation by site (range 80.0%92.9%) (Table 2).
Outcomes by ICD‐9 Diagnosis Code Group and Case‐Identification Algorithm
When compared with discharge diagnosis of fever, adding admission diagnosis of fever captured a higher proportion of hospitalized infants with SBIs (UTI/pyelonephritis, bacteremia/sepsis, or bacterial meningitis). However, median hospital length of stay, severe outcomes, and 3‐day revisits and revisits with hospitalization did not materially differ when including infants with admission diagnosis of fever in addition to discharge diagnosis of fever. Addition of infants with a diagnosis code for serious infection substantially increased the number of infants with SBIs and severe outcomes but did not capture additional 3‐day revisits (Table 3). There were no additional cases of SBI in the no fever/no serious illness diagnosis code group.
ICD‐9 Diagnosis Code Algorithm | Outcome | 3‐Day Revisit, % (95% CI) | 3‐Day Revisit With Hospitalization, % (95% CI) | |||
---|---|---|---|---|---|---|
Hospitalized, % (95% CI) | UTI/Pyelonephritis, Bacteremia/Sepsis, or Bacterial Meningitis, % (95% CI) | Severe Outcome, % (95% CI)* | Length of Stay in Days, Median (IQR) | |||
| ||||||
Discharge diagnosis of fever | 44.3 (40.348.4) | 3.3 (1.84.7) | 1.4 (0.42.3) | 3 (23) | 11.7 (8.215.2) | 5.9 (3.38.4) |
Discharge or admission diagnosis of fever | 52.4 (48.955.9) | 6.1 (4.47.8) | 1.9 (1.02.9) | 3 (23) | 10.9 (7.714.1) | 5.4 (3.17.8) |
Discharge diagnosis of fever or serious infection | 54.0 (50.457.5) | 15.3 (12.717.8) | 3.8 (2.55.2) | 3 (24) | 11.0 (7.714.2) | 5.5 (3.17.9) |
Discharge or admission diagnosis of fever or serious infection | 56.5 (53.259.7) | 12.9 (10.715.1) | 3.6 (2.44.8) | 3 (24) | 10.3 (7.313.3) | 5.2 (3.07.4) |
Among infants who met the reference standard for fever but did not have a discharge or admission diagnosis of fever (false negatives), 11.8% had a diagnosis of SBI. Overall, 43.2% of febrile infants (and 84.4% of hospitalized infants) with SBI did not have an ICD‐9 discharge or admission diagnosis of fever. Addition of ICD‐9 diagnosis codes of serious infection to the algorithm of discharge or admission diagnosis of fever captured all additional SBIs, and no false negativeinfants missed with this algorithm had an SBI.
DISCUSSION
We described the performance of 4 ICD‐9 diagnosis code case‐identification algorithms for the identification of febrile young infants <90 days of age at US children's hospitals. Although the specificity was high across algorithms and institutions, the sensitivity was relatively low, particularly for discharge diagnosis of fever, and varied by institution. Given the high specificity, ICD‐9 diagnosis code case‐identification algorithms for fever reliably identify febrile infants using administrative data with low rates of inclusion of infants without fever. However, underidentification of patients, particularly those more prone to SBIs and severe outcomes depending on the algorithm utilized, can impact interpretation of comparative effectiveness studies or the quality of care delivered by an institution.
ICD‐9 discharge diagnosis codes are frequently used to identify pediatric patients across a variety of administrative databases, diseases, and symptoms.[19, 27, 28, 29, 30, 31] Although discharge diagnosis of fever is highly specific, sensitivity is substantially lower than other case‐identification algorithms we studied, particularly for hospitalized infants. This may be due to a fever code sometimes being omitted in favor of a more specific diagnosis (eg, bacteremia) prior to hospital discharge. Therefore, case identification relying only on ICD‐9 discharge diagnosis codes for fever may under‐report clinically important SBI or severe outcomes as demonstrated in our study. This is in contrast to ICD‐9 diagnosis code identification strategies for childhood UTI and pneumonia, which largely have higher sensitivity but lower specificity than fever codes.[13, 14]
Admission diagnosis of fever is important for febrile infants as they may not have an explicit diagnosis at the time of disposition from the ED. Addition of admission diagnosis of fever to an algorithm relying on discharge diagnosis code alone increased sensitivity without a demonstrable reduction in specificity and PPV, likely due to capture of infants with a fever diagnosis at presentation before a specific infection was identified. Although using an algorithm of discharge or admission diagnosis of fever captured a higher percentage of hospitalized febrile infants with SBIs, sensitivity was only 71% overall with this algorithm, and 43% of febrile infants with SBI would still have been missed. Importantly, though, addition of various ICD‐9 codes for serious infection to this algorithm resulted in capture of all febrile infants with SBI and should be used as a sensitivity analysis.
The test characteristics of diagnosis codes were highest in the 29‐ to 56‐days‐old age group. Given the differing low‐risk criteria[6, 7, 8] and lack of best practice guidelines[16] in this age group, the use of administrative data may allow for the comparison of testing and treatment strategies across a large cohort of febrile infants aged 29 to 56 days. However, individual hospital coding practices may affect algorithm performance, in particular sensitivity, which varied substantially by hospital. This variation in algorithm sensitivity may impact comparisons of outcomes across institutions. Therefore, when conducting studies of febrile infants using administrative data, sensitivity analyses or use of chart review should be considered to augment the use of ICD‐9 code‐based identification strategies, particularly for comparative benchmarking and outcomes studies. These additional analyses are particularly important for studies of febrile infants >56 days of age, in whom the sensitivity of diagnosis codes is particularly low. We speculate that the lower sensitivity in older febrile infants may relate to a lack of consensus on the clinical significance of fever in this age group and the varying management strategies employed.[10]
Strengths of this study include the assessment of ICD‐9 code algorithms across multiple institutions for identification of fever in young infants, and the patterns of our findings remained robust when comparing median performance characteristics of the algorithms across hospitals to our overall findings. We were also able to accurately estimate PPV and NPV using a case‐identification strategy weighted to the actual population sizes. Although sensitivity and specificity are the primary measures of test performance, predictive values are highly informative for investigators using administrative data. Additionally, our findings may inform public health efforts including disease surveillance, assessment of seasonal variation, and identification and monitoring of healthcare‐associated infections among febrile infants.
Our study has limitations. We did not review all identified records, which raises the possibility that our evaluated cohort may not be representative of the entire febrile infant population. We attempted to mitigate this possibility by using a random sampling strategy for our population selection that was weighted to the actual population sizes. Second, we identified serious infections using ICD‐9 diagnosis codes determined by group consensus, which may not capture all serious infection codes that identify febrile infants whose fever code was omitted. Third, 47 infants had abnormal temperature that did not meet our reference standard criteria for fever and were included in the no fever group. Although there may be disagreement regarding what constitutes a fever, we used a widely accepted reference standard to define fever.[16] Further, inclusion of these 47 infants as fever did not materially change algorithm performance. Last, our study was conducted at 8 large tertiary‐care children's hospitals, and our results may not be generalizable to other children's hospitals and community‐based hospitals.
CONCLUSIONS
Studies of febrile young infants that rely on ICD‐9 discharge diagnosis code of fever for case ascertainment have high specificity but low sensitivity for the identification of febrile infants, particularly among hospitalized patients. A case‐identification strategy that includes discharge or admission diagnosis of fever demonstrated higher sensitivity, and should be considered for studies of febrile infants using administrative data. However, additional strategies such as incorporation of ICD‐9 codes for serious infection should be used when comparing outcomes across institutions.
Acknowledgements
The Febrile Young Infant Research Collaborative includes the following additional collaborators who are acknowledged for their work on this study: Erica DiLeo, MA, Department of Medical Education and Research, Danbury Hospital, Danbury, Connecticut; Janet Flores, BS, Division of Emergency Medicine, Ann and Robert H. Lurie Children's Hospital of Chicago, Chicago, Illinois.
Disclosures: This project funded in part by The Gerber Foundation Novice Researcher Award, (Ref No. 1827‐3835). Dr. Fran Balamuth received career development support from the National Institutes of Health (NHLBI K12‐HL109009). Funders were not involved in the design or conduct of the study; collection, management, analysis, or interpretation of the data; or preparation, review, or approval of the manuscript. The authors have no conflicts of interest relevant to this article to disclose.
- The prevalence of serious bacterial infections by age in febrile infants during the first 3 months of life. Pediatr Ann. 1993;22:462–466. .
- Performance of low‐risk criteria in the evaluation of young infants with fever: review of the literature. Pediatrics. 2010;125:228–233. , , .
- A week‐by‐week analysis of the low‐risk criteria for serious bacterial infection in febrile neonates. Arch Dis Child. 2009;94:287–292. , , , , , .
- Is 15 days an appropriate cut‐off age for considering serious bacterial infection in the management of febrile infants? Pediatr Infect Dis J. 2012;31:455–458. , , , et al.
- Failure of infant observation scales in detecting serious illness in febrile, 4‐ to 8‐week‐old infants. Pediatrics. 1990;85:1040–1043. , , .
- Outpatient management without antibiotics of fever in selected infants. N Engl J Med. 1993;329:1437–1441. , , .
- Identifying febrile infants at risk for a serious bacterial infection. J Pediatr. 1993;123:489–490. , , .
- Febrile infants at low risk for serious bacterial infection—an appraisal of the Rochester criteria and implications for management. Febrile Infant Collaborative Study Group. Pediatrics. 1994;94:390–396. , , , et al.
- Management of febrile neonates in US pediatric emergency departments. Pediatrics. 2014;133:187–195. , , , et al.
- Variation in care of the febrile young infant <90 days in US pediatric emergency departments. Pediatrics. 2014;134:667–677. , , , et al.
- Association of clinical practice guidelines with emergency department management of febrile infants ≤56 days of age. J Hosp Med. 2015;10:358–365. , , , et al.
- Diagnosis and management of febrile infants (0‐3 months). Evid Rep Technol Assess (Full Rep). 2012;(205):1–297. , , , et al.
- Accuracy of administrative billing codes to detect urinary tract infection hospitalizations. Pediatrics. 2011;128:323–330. , , , et al.
- Identifying pediatric community‐acquired pneumonia hospitalizations: accuracy of administrative billing codes. JAMA Pediatr. 2013;167:851–858. , , , et al.
- Development and use of reporting guidelines for assessing the quality of validation studies of health administrative data. J Clin Epidemiol. 2011;64:821–829. , , , , , .
- American College of Emergency Physicians Clinical Policies Committee; American College of Emergency Physicians Clinical Policies Subcommittee on Pediatric Fever. Clinical policy for children younger than three years presenting to the emergency department with fever. Ann Emerg Med. 2003;42:530–545.
- Variation in occult injury screening for children with suspected abuse in selected US children's hospitals. Pediatrics. 2012;130:853–860. , , , , , .
- Achieving data quality. How data from a pediatric health information system earns the trust of its users. J AHIMA. 2004;75:22–26. .
- Corticosteroids and mortality in children with bacterial meningitis. JAMA. 2008;299:2048–2055. , , , .
- Research electronic data capture (REDCap)—a metadata‐driven methodology and workflow process for providing translational research informatics support. J Biomed Inform. 2009;42:377–381. , , , , , .
- Deaths attributed to pediatric complex chronic conditions: national trends and implications for supportive care services. Pediatrics. 2001;107:E99. , , , , , .
- US Census Bureau. Geographic terms and concepts—census divisions and census regions. Available at: https://www.census.gov/geo/reference/gtc/gtc_census_divreg.html. Accessed October 20, 2014.
- Initial emergency department diagnosis and return visits: risk versus perception. Ann Emerg Med. 1998;32:569–573. , , , .
- A national depiction of children with return visits to the emergency department within 72 hours, 2001–2007. Pediatr Emerg Care. 2012;28:606–610. , , , , .
- Pediatric observation status: are we overlooking a growing population in children's hospitals? J Hosp Med. 2012;7:530–536. , , , et al.
- Differences in designations of observation care in US freestanding children's hospitals: are they virtual or real? J Hosp Med. 2012;7:287–293. , , , et al.
- Trends in the management of viral meningitis at United States children's hospitals. Pediatrics. 2013;131:670–676. , , , , .
- Impact of increasing ondansetron use on clinical outcomes in children with gastroenteritis. JAMA Pediatr. 2014;168:321–329. , , , et al.
- Race, otitis media, and antibiotic selection. Pediatrics. 2014;134:1059–1066. , , , , .
- Establishing benchmarks for the hospitalized care of children with asthma, bronchiolitis, and pneumonia. Pediatrics. 2014;134:555–562. , , , et al.
- Diagnostic testing and treatment of pediatric headache in the emergency department. J Pediatr. 2013;163:1634–1637. , , , , .
Fever is one of the most common reasons for emergency department (ED) evaluation of infants under 90 days of age.[1] Up to 10% to 20% of febrile young infants will have a serious bacterial infection (SBI),[2, 3, 4] but infants with SBI are difficult to distinguish from those without SBI based upon symptoms and physical examination findings alone.[5] Previously developed clinical prediction algorithms can help to identify febrile infants at low risk for SBI, but differ in age range as well as recommendations for testing and empiric treatment.[6, 7, 8] Consequently, there is widespread variation in management of febrile young infants at US children's hospitals,[9, 10, 11] and defining optimal management strategies remains an important issue in pediatric healthcare.[12] Administrative datasets are convenient and inexpensive, and can be used to evaluate practice variation, trends, and outcomes of a large, diverse group of patients within and across institutions.[9, 10] Accurately identifying febrile infants evaluated for suspected SBI in administrative databases would facilitate comparative effectiveness research, quality improvement initiatives, and institutional benchmarking.
Prior studies have validated the accuracy of administrative billing codes for identification of other common childhood illnesses, including urinary tract infection (UTI)[13] and pneumonia.[14] The accuracy of International Classification of Diseases, Ninth Revision (ICD‐9) diagnosis codes in identifying febrile young infants evaluated for SBI is not known. Reliance on administrative ICD‐9 diagnosis codes for patient identification can lead to misclassification of patients due to variable database quality, the validity of the diagnosis codes being utilized, and hospital coding practices.[15] Additionally, fever is a symptom and not a specific diagnosis. If a particular bacterial or viral diagnosis is established (eg, enterovirus meningitis), a discharge diagnosis of fever may not be attributed to the patient encounter. Thus, evaluating the performance characteristics and capture of clinical outcomes of different combinations of ICD‐9 diagnosis codes for identifying febrile infants is necessary for both the conduct and interpretation of studies that utilize administrative databases. The primary objective of this investigation was to identify the most accurate ICD‐9 coding strategies for the identification of febrile infants aged <90 days using administrative data. We also sought to evaluate capture of clinically important outcomes across identification strategies.
METHODS
Study Design and Setting
For this multicenter retrospective study, we used the Pediatric Health Information System (PHIS) database to identify infants <90 days of age[16] who presented between July 1, 2012 and June 30, 2013 to 1 of 8 EDs. We assessed performance characteristics of ICD‐9 diagnosis code case‐identification algorithms by comparing ICD‐9 code combinations to a fever reference standard determined by medical record review. The institutional review board at each participating site approved the study protocol.
Data Source
Data were obtained from 2 sources: the PHIS database and medical record review. We used the PHIS database to identify eligible patients by ICD‐9 diagnosis codes; patient encounters were randomly selected using a random number generator. The PHIS database contains demographic, diagnosis, and billing data from 44 hospitals affiliated with the Children's Hospital Association (Overland Park, Kansas) and represents 85% of freestanding children's hospitals in the United States.[17] Data are deidentified; encrypted unique patient identifiers permit tracking of patients across visits within a site.[18] The Children's Hospital Association and participating hospitals jointly assure the quality and integrity of the data.[19]
For each patient encounter identified in the PHIS database, detailed medical record review was performed by trained investigators at each of the 8 study sites (see Supporting Information, Appendix, in the online version of this article). A standardized data collection instrument was pilot tested by all investigators prior to use. Data were collected and managed using the Research Electronic Data Capture (REDCap) tool hosted at Boston Children's Hospital.[20]
Exclusions
Using PHIS data, prior to medical record review we excluded infants with a complex chronic condition as defined previously[21] and those transferred from another institution, as these infants may warrant a nonstandard evaluation and/or may have incomplete data.
ICD‐9 Diagnosis Code Groups
In the PHIS database, all patients discharged from the hospital (including hospitalized patients as well as patients discharged from the ED) receive 1 or more ICD‐9 discharge diagnosis codes. These diagnosis codes are ascribed after discharge from the hospital, or for ED patients, after ED discharge. Additionally, patients may receive an admission diagnosis, which reflects the diagnosis ascribed at the time of ED discharge or transfer to the inpatient unit.
We reviewed medical records of infants selected from the following ICD‐9 diagnosis code groups (Figure 1): (1) discharge diagnosis code of fever (780.6 [fever and other physiologic disturbances of temperature regulation], 778.4 [other disturbances of temperature regulation of newborn], 780.60 [fever, unspecified], or 780.61 [fever presenting with conditions classified elsewhere])[9, 10] regardless of the presence of admission diagnosis of fever or diagnosis of serious infection, (2) admission diagnosis code of fever without associated discharge diagnosis code of fever,[10] (3) discharge diagnosis code of serious infection determined a priori (see Supporting Information, Appendix, in the online version of this article) without discharge or admission diagnosis code of fever, and (4) infants without any diagnosis code of fever or serious infection.
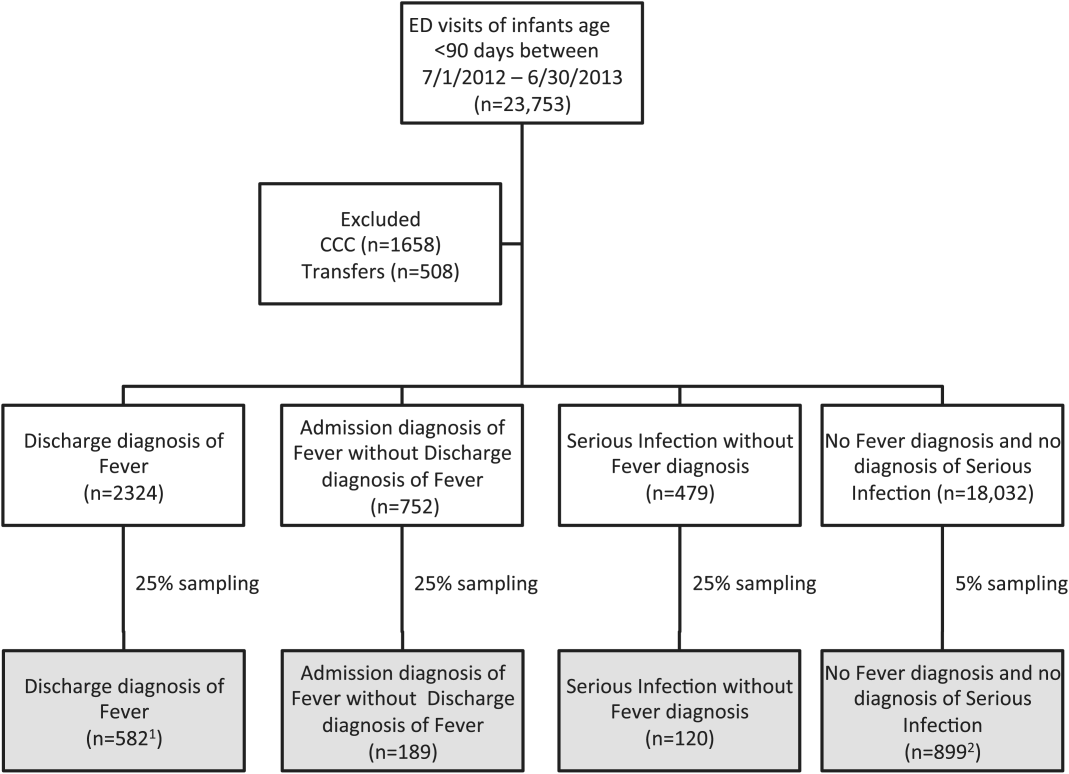
Medical records reviewed in each of the 4 ICD‐9 diagnosis code groups were randomly selected from the overall set of ED encounters in the population of infants <90 days of age evaluated during the study period. Twenty‐five percent population sampling was used for 3 of the ICD‐9 diagnosis code groups, whereas 5% sampling was used for the no fever/no serious infection code group. The number of medical records reviewed in each ICD‐9 diagnosis code group was proportional to the distribution of ICD‐9 codes across the entire population of infants <90 days of age. These records were distributed equally across sites (228 records per site), except for 1 site that does not assign admission diagnoses (201 records).
Investigators were blinded to ICD‐9 diagnosis code groups during medical record review. Infants with multiple visits during the study period were eligible to be included more than once if the visits occurred more than 3 days apart. For infants with more than 1 ED visit on a particular calendar day, investigators were instructed to review the initial visit.
For each encounter, we also abstracted demographic characteristics (gender, race/ethnicity), insurance status, hospital region (using US Census categories[22]), and season from the PHIS database.
Reference Standard
The presence of fever was determined by medical record review. We defined fever as any documented temperature 100.4F (38.0C) at home or in the ED.[16]
ICD‐9 Code Case‐Identification Algorithms
Using the aforementioned ICD‐9 diagnosis code groups individually and in combination, the following 4 case‐identification algorithms, determined from prior study or group consensus, were compared to the reference standard: (1) ICD‐9 discharge diagnosis code of fever,[9] (2) ICD‐9 admission or discharge diagnosis code of fever,[10, 11] (3) ICD‐9 discharge diagnosis code of fever or serious infection, and (4) ICD‐9 discharge or admission diagnosis code of fever or serious infection. Algorithms were compared overall, separately for discharged and hospitalized infants, and across 3 distinct age groups (28 days, 2956 days, and 5789 days).
Patient‐Level Outcomes
To compare differences in outcomes by case‐identification algorithm, from the PHIS database we abstracted hospitalization rates, rates of UTI/pyelonephritis,[13] bacteremia/sepsis, and bacterial meningitis.[19] Severe outcomes were defined as intensive care unit admission, mechanical ventilation, central line placement, receipt of extracorporeal membrane oxygenation, or death. We assessed hospital length of stay for admitted infants and 3‐day revisits,[23, 24] and revisits resulting in hospitalization for infants discharged from the ED at the index visit. Patients billed for observation care were classified as being hospitalized.[25, 26]
Data Analysis
Accuracy of the 4 case‐identification algorithms (compared with the reference standard) was calculated using sensitivity, specificity, negative predictive value (NPV), and positive predictive value (PPV), along with 95% confidence interval (CI). Prior to analysis, a 5‐fold weighting factor was applied to the no fever/no serious infection group to account for the differential sampling used for this group (5% vs 25% for the other 3 ICD‐9 diagnosis code groups). This weighting was done to approximate the true prevalence of each ICD‐9 code group within the larger population, so that an accurate rate of false negatives (infants with fever who had neither a diagnosis of fever nor serious infection) could be calculated.
We described continuous variables using median and interquartile range or range values and categorical variables using frequencies with 95% CIs. We compared categorical variables using a 2 test. We determined statistical significance as a 2‐tailed P value <0.05. Statistical analyses were performed using SAS version 9.3 (SAS Institute, Cary, NC).
RESULTS
Study Patients
During the 1‐year study period, 23,753 ED encounters for infants <90 days of age were identified in the PHIS database at the 8 participating sites. Of these infant encounters, 2166 (9.2%) were excluded (1658 infants who had a complex chronic condition and 508 transferred into the ED), leaving 21,587 infants available for selection. After applying our sampling strategy, we identified 1797 encounters for medical record review. Seven encounters from 3 hospitals with missing medical records were excluded, resulting in a final cohort of 1790 encounters (Figure 1). Among included infants, 552 (30.8%) were 28 days, 743 (41.5%) were 29 to 56 days, and 495 (27.8%) were 57 to 89 days of age; 737 (41.2%) infants were hospitalized. Patients differed in age, race, payer, and season across ICD‐9 diagnosis code groups (see Supporting Information, Table 1, in the online version of this article).
ICD‐9 Diagnosis Code Algorithm | Overall | |||
---|---|---|---|---|
Sensitivity, % (95% CI) | Specificity, % (95% CI) | Negative Predictive Value, % (95% CI) | Positive Predictive Value, % (95% CI) | |
| ||||
Discharge diagnosis of fever | 53.2 (50.056.4) | 98.2 (97.898.6) | 90.8 (90.091.6) | 86.1 (83.388.9) |
Hospitalized | 47.3 (43.151.5) | 97.7 (96.998.5) | 80.6 (78.682.6) | 90.2 (86.893.6) |
Discharged from ED | 61.4 (56.666.2) | 98.4 (98.098.8) | 95.4 (94.796.1) | 82.1 (77.786.5) |
Discharge or admission diagnosis of Fever | 71.1 (68.274.0) | 97.7 (97.398.1) | 94.1 (93.494.8) | 86.9 (84.589.3) |
Hospitalized | 72.5 (68.876.2) | 97.1 (96.298.0) | 88.8 (87.190.5) | 91.7 (89.194.3) |
Discharged from ED | 69.2 (64.773.7) | 98.0 (97.598.5) | 96.3 (95.796.9) | 80.8 (76.685.0) |
Discharge diagnosis of fever or serious infection | 63.7 (60.666.8) | 96.5 (96.097.0) | 92.6 (91.893.4) | 79.6 (76.782.5) |
Hospitalized | 63.9 (59.967.9) | 92.5 (91.094.0) | 85.1 (83.287.0) | 79.1 (75.382.9) |
Discharged from ED | 63.4 (58.768.1) | 98.1 (97.698.6) | 95.6 (94.996.3) | 80.2 (75.884.6) |
Discharge or admission diagnosis of fever or serious infection | 76.6 (73.979.3) | 96.2 (95.696.8) | 95.1 (94.595.7) | 81.0 (78.483.6) |
Hospitalized | 80.8 (77.584.1) | 92.1 (90.693.6) | 91.5 (89.993.1) | 82.1 (78.985.3) |
Discharged from ED | 71.0 (66.575.5) | 97.7 (97.298.2) | 96.5 (95.997.1) | 79.4 (75.283.6) |
Among the 1790 patient encounters reviewed, a total of 766 infants (42.8%) met the reference standard definition for fever in the cohort. An additional 47 infants had abnormal temperature reported (documentation of tactile fever, history of fever without a specific temperature described, or hypothermia) but were classified as having no fever by the reference standard.
ICD‐9 Code Case‐Identification Algorithm Performance
Compared with the reference standard, the 4 case‐identification algorithms demonstrated specificity of 96.2% to 98.2% but lower sensitivity overall (Figure 2). Discharge diagnosis of fever alone demonstrated the lowest sensitivity. The algorithm of discharge or admission diagnosis of fever resulted in increased sensitivity and the highest PPV of all 4 algorithms (86.9%, 95% CI: 84.5‐89.3). Addition of serious infection codes to this algorithm resulted in a marginal increase in sensitivity and a similar decrease in PPV (Table 1). When limited to hospitalized infants, specificity was highest for the case‐identification algorithm of discharge diagnosis of fever and similarly high for discharge or admission diagnosis of fever; sensitivity was highest for the algorithm of discharge or admission diagnosis of fever or diagnosis of serious infection. For infants discharged from the ED, algorithm specificity was 97.7% to 98.4%, with lower sensitivity for all 4 algorithms (Table 1). Inclusion of the 47 infants with abnormal temperature as fever did not materially change algorithm performance (data not shown).
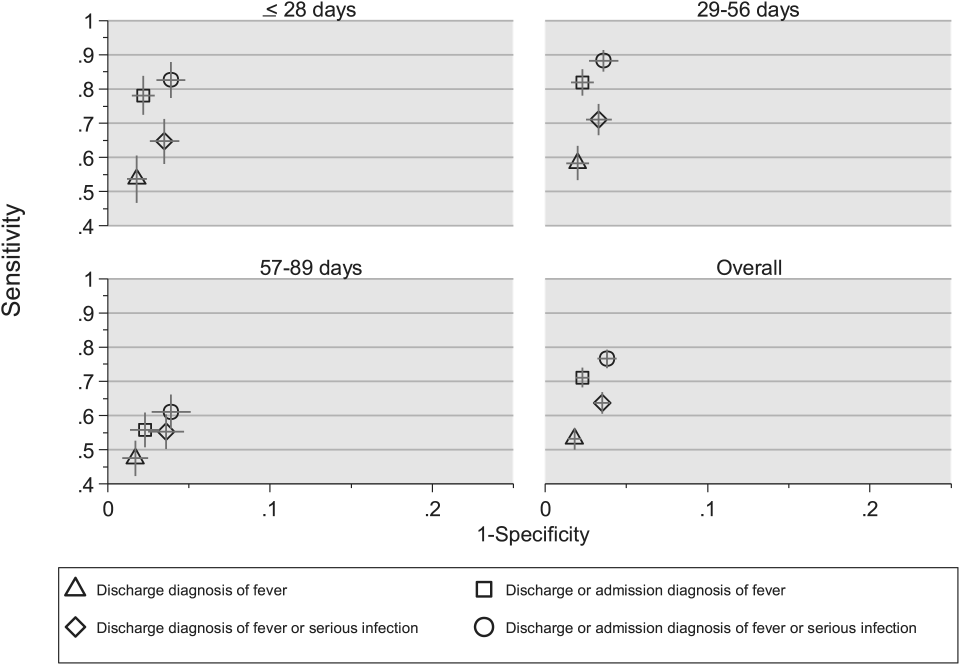
Across all 3 age groups (28 days, 2956 days, and 5789 days), the 4 case‐identification algorithms demonstrated specificity >96%, whereas algorithm sensitivity was highest in the 29‐ to 56‐days‐old age group and lowest among infants 57 to 89 days old across all 4 algorithms (Figure 2). Similar to the overall cohort, an algorithm of discharge or admission diagnosis of fever demonstrated specificity of nearly 98% in all age groups; addition of serious infection codes to this algorithm increased sensitivity, highest in the 29‐ to 56‐days‐old age group (Figure 2; see also Supporting Information, Table 2, in the online version of this article).
ICD‐9 Diagnosis Code Algorithm | Sensitivity, Median % (Range) | Specificity, Median % (Range) | Negative Predictive Value, Median % (Range) | Positive Predictive Value, Median % (Range) |
---|---|---|---|---|
| ||||
Discharge diagnosis of fever | 56.2 (34.681.0) | 98.3 (96.499.1) | 92.1 (83.297.4) | 87.7 (74.093.2) |
Discharge or Admission diagnosis of Fever | 76.7 (51.385.0) | 97.8 (96.298.7) | 95.6 (86.997.4) | 87.4 (80.092.9) |
Discharge diagnosis of fever or serious infection | 68.3 (44.287.3) | 96.5 (95.498.0) | 93.6 (85.298.2) | 78.3 (74.289.0) |
Discharge or admission diagnosis of fever or serious infection | 83.1 (58.390.7) | 95.8 (95.498.0) | 96.5 (88.598.2) | 79.1 (77.490.4) |
Across the 8 study sites, median specificity was 95.8% to 98.3% for the 4 algorithms, with little interhospital variability; however, algorithm sensitivity varied widely by site. Median PPV was highest for discharge diagnosis of fever alone at 87.7% but ranged from 74.0% to 93.2% across sites. Median PPV for an algorithm of discharge or admission diagnosis of fever was similar (87.4%) but with less variation by site (range 80.0%92.9%) (Table 2).
Outcomes by ICD‐9 Diagnosis Code Group and Case‐Identification Algorithm
When compared with discharge diagnosis of fever, adding admission diagnosis of fever captured a higher proportion of hospitalized infants with SBIs (UTI/pyelonephritis, bacteremia/sepsis, or bacterial meningitis). However, median hospital length of stay, severe outcomes, and 3‐day revisits and revisits with hospitalization did not materially differ when including infants with admission diagnosis of fever in addition to discharge diagnosis of fever. Addition of infants with a diagnosis code for serious infection substantially increased the number of infants with SBIs and severe outcomes but did not capture additional 3‐day revisits (Table 3). There were no additional cases of SBI in the no fever/no serious illness diagnosis code group.
ICD‐9 Diagnosis Code Algorithm | Outcome | 3‐Day Revisit, % (95% CI) | 3‐Day Revisit With Hospitalization, % (95% CI) | |||
---|---|---|---|---|---|---|
Hospitalized, % (95% CI) | UTI/Pyelonephritis, Bacteremia/Sepsis, or Bacterial Meningitis, % (95% CI) | Severe Outcome, % (95% CI)* | Length of Stay in Days, Median (IQR) | |||
| ||||||
Discharge diagnosis of fever | 44.3 (40.348.4) | 3.3 (1.84.7) | 1.4 (0.42.3) | 3 (23) | 11.7 (8.215.2) | 5.9 (3.38.4) |
Discharge or admission diagnosis of fever | 52.4 (48.955.9) | 6.1 (4.47.8) | 1.9 (1.02.9) | 3 (23) | 10.9 (7.714.1) | 5.4 (3.17.8) |
Discharge diagnosis of fever or serious infection | 54.0 (50.457.5) | 15.3 (12.717.8) | 3.8 (2.55.2) | 3 (24) | 11.0 (7.714.2) | 5.5 (3.17.9) |
Discharge or admission diagnosis of fever or serious infection | 56.5 (53.259.7) | 12.9 (10.715.1) | 3.6 (2.44.8) | 3 (24) | 10.3 (7.313.3) | 5.2 (3.07.4) |
Among infants who met the reference standard for fever but did not have a discharge or admission diagnosis of fever (false negatives), 11.8% had a diagnosis of SBI. Overall, 43.2% of febrile infants (and 84.4% of hospitalized infants) with SBI did not have an ICD‐9 discharge or admission diagnosis of fever. Addition of ICD‐9 diagnosis codes of serious infection to the algorithm of discharge or admission diagnosis of fever captured all additional SBIs, and no false negativeinfants missed with this algorithm had an SBI.
DISCUSSION
We described the performance of 4 ICD‐9 diagnosis code case‐identification algorithms for the identification of febrile young infants <90 days of age at US children's hospitals. Although the specificity was high across algorithms and institutions, the sensitivity was relatively low, particularly for discharge diagnosis of fever, and varied by institution. Given the high specificity, ICD‐9 diagnosis code case‐identification algorithms for fever reliably identify febrile infants using administrative data with low rates of inclusion of infants without fever. However, underidentification of patients, particularly those more prone to SBIs and severe outcomes depending on the algorithm utilized, can impact interpretation of comparative effectiveness studies or the quality of care delivered by an institution.
ICD‐9 discharge diagnosis codes are frequently used to identify pediatric patients across a variety of administrative databases, diseases, and symptoms.[19, 27, 28, 29, 30, 31] Although discharge diagnosis of fever is highly specific, sensitivity is substantially lower than other case‐identification algorithms we studied, particularly for hospitalized infants. This may be due to a fever code sometimes being omitted in favor of a more specific diagnosis (eg, bacteremia) prior to hospital discharge. Therefore, case identification relying only on ICD‐9 discharge diagnosis codes for fever may under‐report clinically important SBI or severe outcomes as demonstrated in our study. This is in contrast to ICD‐9 diagnosis code identification strategies for childhood UTI and pneumonia, which largely have higher sensitivity but lower specificity than fever codes.[13, 14]
Admission diagnosis of fever is important for febrile infants as they may not have an explicit diagnosis at the time of disposition from the ED. Addition of admission diagnosis of fever to an algorithm relying on discharge diagnosis code alone increased sensitivity without a demonstrable reduction in specificity and PPV, likely due to capture of infants with a fever diagnosis at presentation before a specific infection was identified. Although using an algorithm of discharge or admission diagnosis of fever captured a higher percentage of hospitalized febrile infants with SBIs, sensitivity was only 71% overall with this algorithm, and 43% of febrile infants with SBI would still have been missed. Importantly, though, addition of various ICD‐9 codes for serious infection to this algorithm resulted in capture of all febrile infants with SBI and should be used as a sensitivity analysis.
The test characteristics of diagnosis codes were highest in the 29‐ to 56‐days‐old age group. Given the differing low‐risk criteria[6, 7, 8] and lack of best practice guidelines[16] in this age group, the use of administrative data may allow for the comparison of testing and treatment strategies across a large cohort of febrile infants aged 29 to 56 days. However, individual hospital coding practices may affect algorithm performance, in particular sensitivity, which varied substantially by hospital. This variation in algorithm sensitivity may impact comparisons of outcomes across institutions. Therefore, when conducting studies of febrile infants using administrative data, sensitivity analyses or use of chart review should be considered to augment the use of ICD‐9 code‐based identification strategies, particularly for comparative benchmarking and outcomes studies. These additional analyses are particularly important for studies of febrile infants >56 days of age, in whom the sensitivity of diagnosis codes is particularly low. We speculate that the lower sensitivity in older febrile infants may relate to a lack of consensus on the clinical significance of fever in this age group and the varying management strategies employed.[10]
Strengths of this study include the assessment of ICD‐9 code algorithms across multiple institutions for identification of fever in young infants, and the patterns of our findings remained robust when comparing median performance characteristics of the algorithms across hospitals to our overall findings. We were also able to accurately estimate PPV and NPV using a case‐identification strategy weighted to the actual population sizes. Although sensitivity and specificity are the primary measures of test performance, predictive values are highly informative for investigators using administrative data. Additionally, our findings may inform public health efforts including disease surveillance, assessment of seasonal variation, and identification and monitoring of healthcare‐associated infections among febrile infants.
Our study has limitations. We did not review all identified records, which raises the possibility that our evaluated cohort may not be representative of the entire febrile infant population. We attempted to mitigate this possibility by using a random sampling strategy for our population selection that was weighted to the actual population sizes. Second, we identified serious infections using ICD‐9 diagnosis codes determined by group consensus, which may not capture all serious infection codes that identify febrile infants whose fever code was omitted. Third, 47 infants had abnormal temperature that did not meet our reference standard criteria for fever and were included in the no fever group. Although there may be disagreement regarding what constitutes a fever, we used a widely accepted reference standard to define fever.[16] Further, inclusion of these 47 infants as fever did not materially change algorithm performance. Last, our study was conducted at 8 large tertiary‐care children's hospitals, and our results may not be generalizable to other children's hospitals and community‐based hospitals.
CONCLUSIONS
Studies of febrile young infants that rely on ICD‐9 discharge diagnosis code of fever for case ascertainment have high specificity but low sensitivity for the identification of febrile infants, particularly among hospitalized patients. A case‐identification strategy that includes discharge or admission diagnosis of fever demonstrated higher sensitivity, and should be considered for studies of febrile infants using administrative data. However, additional strategies such as incorporation of ICD‐9 codes for serious infection should be used when comparing outcomes across institutions.
Acknowledgements
The Febrile Young Infant Research Collaborative includes the following additional collaborators who are acknowledged for their work on this study: Erica DiLeo, MA, Department of Medical Education and Research, Danbury Hospital, Danbury, Connecticut; Janet Flores, BS, Division of Emergency Medicine, Ann and Robert H. Lurie Children's Hospital of Chicago, Chicago, Illinois.
Disclosures: This project funded in part by The Gerber Foundation Novice Researcher Award, (Ref No. 1827‐3835). Dr. Fran Balamuth received career development support from the National Institutes of Health (NHLBI K12‐HL109009). Funders were not involved in the design or conduct of the study; collection, management, analysis, or interpretation of the data; or preparation, review, or approval of the manuscript. The authors have no conflicts of interest relevant to this article to disclose.
Fever is one of the most common reasons for emergency department (ED) evaluation of infants under 90 days of age.[1] Up to 10% to 20% of febrile young infants will have a serious bacterial infection (SBI),[2, 3, 4] but infants with SBI are difficult to distinguish from those without SBI based upon symptoms and physical examination findings alone.[5] Previously developed clinical prediction algorithms can help to identify febrile infants at low risk for SBI, but differ in age range as well as recommendations for testing and empiric treatment.[6, 7, 8] Consequently, there is widespread variation in management of febrile young infants at US children's hospitals,[9, 10, 11] and defining optimal management strategies remains an important issue in pediatric healthcare.[12] Administrative datasets are convenient and inexpensive, and can be used to evaluate practice variation, trends, and outcomes of a large, diverse group of patients within and across institutions.[9, 10] Accurately identifying febrile infants evaluated for suspected SBI in administrative databases would facilitate comparative effectiveness research, quality improvement initiatives, and institutional benchmarking.
Prior studies have validated the accuracy of administrative billing codes for identification of other common childhood illnesses, including urinary tract infection (UTI)[13] and pneumonia.[14] The accuracy of International Classification of Diseases, Ninth Revision (ICD‐9) diagnosis codes in identifying febrile young infants evaluated for SBI is not known. Reliance on administrative ICD‐9 diagnosis codes for patient identification can lead to misclassification of patients due to variable database quality, the validity of the diagnosis codes being utilized, and hospital coding practices.[15] Additionally, fever is a symptom and not a specific diagnosis. If a particular bacterial or viral diagnosis is established (eg, enterovirus meningitis), a discharge diagnosis of fever may not be attributed to the patient encounter. Thus, evaluating the performance characteristics and capture of clinical outcomes of different combinations of ICD‐9 diagnosis codes for identifying febrile infants is necessary for both the conduct and interpretation of studies that utilize administrative databases. The primary objective of this investigation was to identify the most accurate ICD‐9 coding strategies for the identification of febrile infants aged <90 days using administrative data. We also sought to evaluate capture of clinically important outcomes across identification strategies.
METHODS
Study Design and Setting
For this multicenter retrospective study, we used the Pediatric Health Information System (PHIS) database to identify infants <90 days of age[16] who presented between July 1, 2012 and June 30, 2013 to 1 of 8 EDs. We assessed performance characteristics of ICD‐9 diagnosis code case‐identification algorithms by comparing ICD‐9 code combinations to a fever reference standard determined by medical record review. The institutional review board at each participating site approved the study protocol.
Data Source
Data were obtained from 2 sources: the PHIS database and medical record review. We used the PHIS database to identify eligible patients by ICD‐9 diagnosis codes; patient encounters were randomly selected using a random number generator. The PHIS database contains demographic, diagnosis, and billing data from 44 hospitals affiliated with the Children's Hospital Association (Overland Park, Kansas) and represents 85% of freestanding children's hospitals in the United States.[17] Data are deidentified; encrypted unique patient identifiers permit tracking of patients across visits within a site.[18] The Children's Hospital Association and participating hospitals jointly assure the quality and integrity of the data.[19]
For each patient encounter identified in the PHIS database, detailed medical record review was performed by trained investigators at each of the 8 study sites (see Supporting Information, Appendix, in the online version of this article). A standardized data collection instrument was pilot tested by all investigators prior to use. Data were collected and managed using the Research Electronic Data Capture (REDCap) tool hosted at Boston Children's Hospital.[20]
Exclusions
Using PHIS data, prior to medical record review we excluded infants with a complex chronic condition as defined previously[21] and those transferred from another institution, as these infants may warrant a nonstandard evaluation and/or may have incomplete data.
ICD‐9 Diagnosis Code Groups
In the PHIS database, all patients discharged from the hospital (including hospitalized patients as well as patients discharged from the ED) receive 1 or more ICD‐9 discharge diagnosis codes. These diagnosis codes are ascribed after discharge from the hospital, or for ED patients, after ED discharge. Additionally, patients may receive an admission diagnosis, which reflects the diagnosis ascribed at the time of ED discharge or transfer to the inpatient unit.
We reviewed medical records of infants selected from the following ICD‐9 diagnosis code groups (Figure 1): (1) discharge diagnosis code of fever (780.6 [fever and other physiologic disturbances of temperature regulation], 778.4 [other disturbances of temperature regulation of newborn], 780.60 [fever, unspecified], or 780.61 [fever presenting with conditions classified elsewhere])[9, 10] regardless of the presence of admission diagnosis of fever or diagnosis of serious infection, (2) admission diagnosis code of fever without associated discharge diagnosis code of fever,[10] (3) discharge diagnosis code of serious infection determined a priori (see Supporting Information, Appendix, in the online version of this article) without discharge or admission diagnosis code of fever, and (4) infants without any diagnosis code of fever or serious infection.
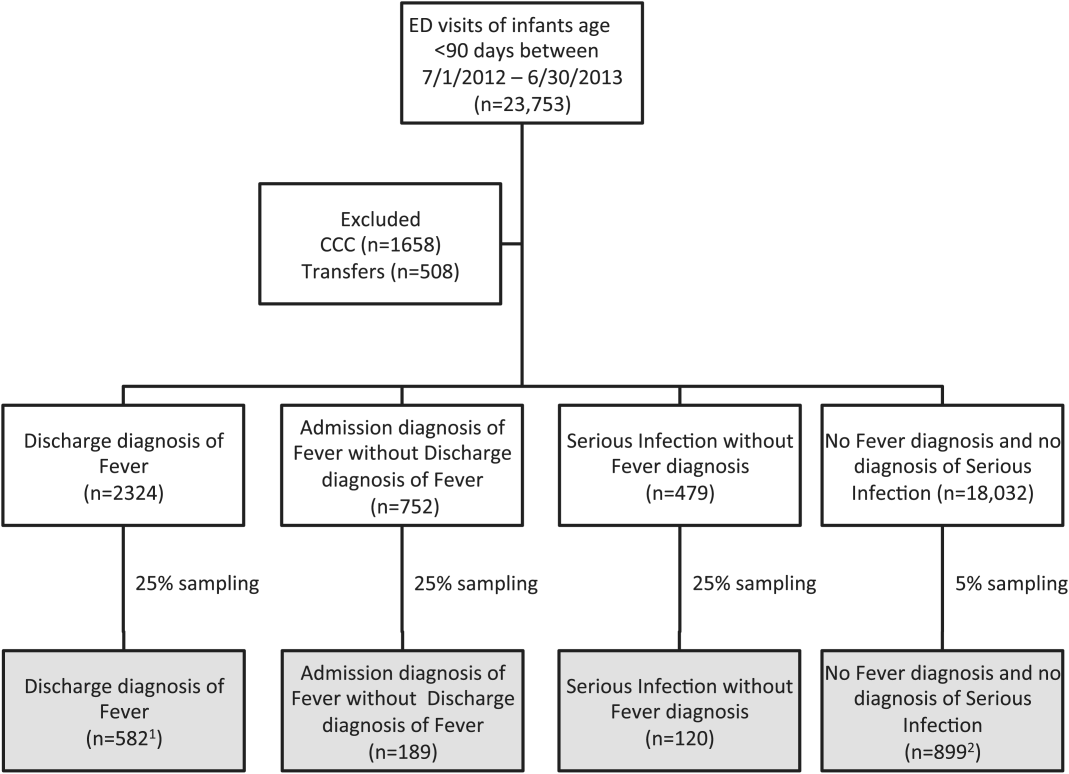
Medical records reviewed in each of the 4 ICD‐9 diagnosis code groups were randomly selected from the overall set of ED encounters in the population of infants <90 days of age evaluated during the study period. Twenty‐five percent population sampling was used for 3 of the ICD‐9 diagnosis code groups, whereas 5% sampling was used for the no fever/no serious infection code group. The number of medical records reviewed in each ICD‐9 diagnosis code group was proportional to the distribution of ICD‐9 codes across the entire population of infants <90 days of age. These records were distributed equally across sites (228 records per site), except for 1 site that does not assign admission diagnoses (201 records).
Investigators were blinded to ICD‐9 diagnosis code groups during medical record review. Infants with multiple visits during the study period were eligible to be included more than once if the visits occurred more than 3 days apart. For infants with more than 1 ED visit on a particular calendar day, investigators were instructed to review the initial visit.
For each encounter, we also abstracted demographic characteristics (gender, race/ethnicity), insurance status, hospital region (using US Census categories[22]), and season from the PHIS database.
Reference Standard
The presence of fever was determined by medical record review. We defined fever as any documented temperature 100.4F (38.0C) at home or in the ED.[16]
ICD‐9 Code Case‐Identification Algorithms
Using the aforementioned ICD‐9 diagnosis code groups individually and in combination, the following 4 case‐identification algorithms, determined from prior study or group consensus, were compared to the reference standard: (1) ICD‐9 discharge diagnosis code of fever,[9] (2) ICD‐9 admission or discharge diagnosis code of fever,[10, 11] (3) ICD‐9 discharge diagnosis code of fever or serious infection, and (4) ICD‐9 discharge or admission diagnosis code of fever or serious infection. Algorithms were compared overall, separately for discharged and hospitalized infants, and across 3 distinct age groups (28 days, 2956 days, and 5789 days).
Patient‐Level Outcomes
To compare differences in outcomes by case‐identification algorithm, from the PHIS database we abstracted hospitalization rates, rates of UTI/pyelonephritis,[13] bacteremia/sepsis, and bacterial meningitis.[19] Severe outcomes were defined as intensive care unit admission, mechanical ventilation, central line placement, receipt of extracorporeal membrane oxygenation, or death. We assessed hospital length of stay for admitted infants and 3‐day revisits,[23, 24] and revisits resulting in hospitalization for infants discharged from the ED at the index visit. Patients billed for observation care were classified as being hospitalized.[25, 26]
Data Analysis
Accuracy of the 4 case‐identification algorithms (compared with the reference standard) was calculated using sensitivity, specificity, negative predictive value (NPV), and positive predictive value (PPV), along with 95% confidence interval (CI). Prior to analysis, a 5‐fold weighting factor was applied to the no fever/no serious infection group to account for the differential sampling used for this group (5% vs 25% for the other 3 ICD‐9 diagnosis code groups). This weighting was done to approximate the true prevalence of each ICD‐9 code group within the larger population, so that an accurate rate of false negatives (infants with fever who had neither a diagnosis of fever nor serious infection) could be calculated.
We described continuous variables using median and interquartile range or range values and categorical variables using frequencies with 95% CIs. We compared categorical variables using a 2 test. We determined statistical significance as a 2‐tailed P value <0.05. Statistical analyses were performed using SAS version 9.3 (SAS Institute, Cary, NC).
RESULTS
Study Patients
During the 1‐year study period, 23,753 ED encounters for infants <90 days of age were identified in the PHIS database at the 8 participating sites. Of these infant encounters, 2166 (9.2%) were excluded (1658 infants who had a complex chronic condition and 508 transferred into the ED), leaving 21,587 infants available for selection. After applying our sampling strategy, we identified 1797 encounters for medical record review. Seven encounters from 3 hospitals with missing medical records were excluded, resulting in a final cohort of 1790 encounters (Figure 1). Among included infants, 552 (30.8%) were 28 days, 743 (41.5%) were 29 to 56 days, and 495 (27.8%) were 57 to 89 days of age; 737 (41.2%) infants were hospitalized. Patients differed in age, race, payer, and season across ICD‐9 diagnosis code groups (see Supporting Information, Table 1, in the online version of this article).
ICD‐9 Diagnosis Code Algorithm | Overall | |||
---|---|---|---|---|
Sensitivity, % (95% CI) | Specificity, % (95% CI) | Negative Predictive Value, % (95% CI) | Positive Predictive Value, % (95% CI) | |
| ||||
Discharge diagnosis of fever | 53.2 (50.056.4) | 98.2 (97.898.6) | 90.8 (90.091.6) | 86.1 (83.388.9) |
Hospitalized | 47.3 (43.151.5) | 97.7 (96.998.5) | 80.6 (78.682.6) | 90.2 (86.893.6) |
Discharged from ED | 61.4 (56.666.2) | 98.4 (98.098.8) | 95.4 (94.796.1) | 82.1 (77.786.5) |
Discharge or admission diagnosis of Fever | 71.1 (68.274.0) | 97.7 (97.398.1) | 94.1 (93.494.8) | 86.9 (84.589.3) |
Hospitalized | 72.5 (68.876.2) | 97.1 (96.298.0) | 88.8 (87.190.5) | 91.7 (89.194.3) |
Discharged from ED | 69.2 (64.773.7) | 98.0 (97.598.5) | 96.3 (95.796.9) | 80.8 (76.685.0) |
Discharge diagnosis of fever or serious infection | 63.7 (60.666.8) | 96.5 (96.097.0) | 92.6 (91.893.4) | 79.6 (76.782.5) |
Hospitalized | 63.9 (59.967.9) | 92.5 (91.094.0) | 85.1 (83.287.0) | 79.1 (75.382.9) |
Discharged from ED | 63.4 (58.768.1) | 98.1 (97.698.6) | 95.6 (94.996.3) | 80.2 (75.884.6) |
Discharge or admission diagnosis of fever or serious infection | 76.6 (73.979.3) | 96.2 (95.696.8) | 95.1 (94.595.7) | 81.0 (78.483.6) |
Hospitalized | 80.8 (77.584.1) | 92.1 (90.693.6) | 91.5 (89.993.1) | 82.1 (78.985.3) |
Discharged from ED | 71.0 (66.575.5) | 97.7 (97.298.2) | 96.5 (95.997.1) | 79.4 (75.283.6) |
Among the 1790 patient encounters reviewed, a total of 766 infants (42.8%) met the reference standard definition for fever in the cohort. An additional 47 infants had abnormal temperature reported (documentation of tactile fever, history of fever without a specific temperature described, or hypothermia) but were classified as having no fever by the reference standard.
ICD‐9 Code Case‐Identification Algorithm Performance
Compared with the reference standard, the 4 case‐identification algorithms demonstrated specificity of 96.2% to 98.2% but lower sensitivity overall (Figure 2). Discharge diagnosis of fever alone demonstrated the lowest sensitivity. The algorithm of discharge or admission diagnosis of fever resulted in increased sensitivity and the highest PPV of all 4 algorithms (86.9%, 95% CI: 84.5‐89.3). Addition of serious infection codes to this algorithm resulted in a marginal increase in sensitivity and a similar decrease in PPV (Table 1). When limited to hospitalized infants, specificity was highest for the case‐identification algorithm of discharge diagnosis of fever and similarly high for discharge or admission diagnosis of fever; sensitivity was highest for the algorithm of discharge or admission diagnosis of fever or diagnosis of serious infection. For infants discharged from the ED, algorithm specificity was 97.7% to 98.4%, with lower sensitivity for all 4 algorithms (Table 1). Inclusion of the 47 infants with abnormal temperature as fever did not materially change algorithm performance (data not shown).
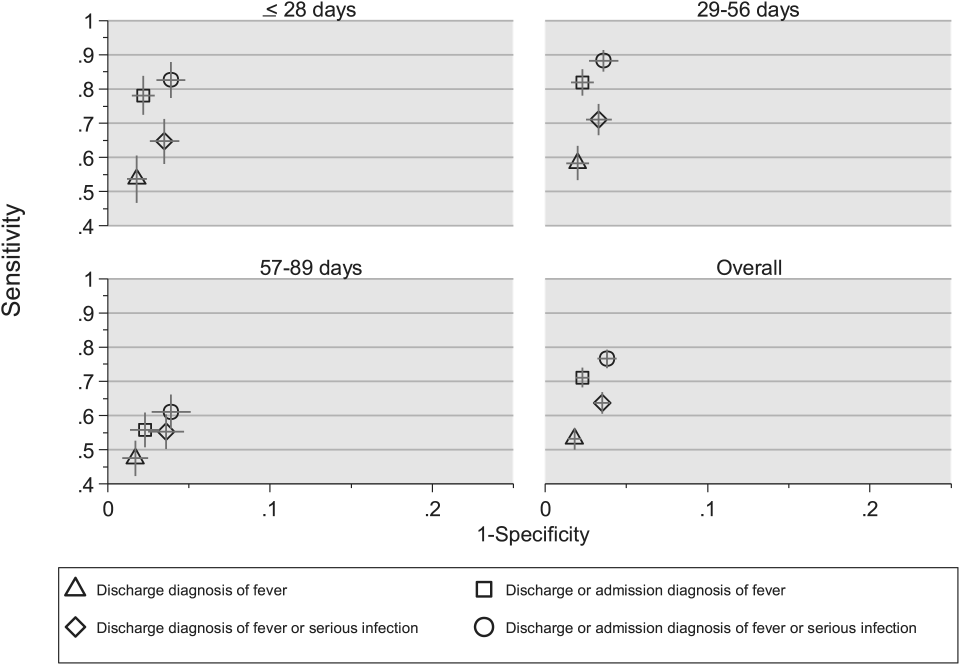
Across all 3 age groups (28 days, 2956 days, and 5789 days), the 4 case‐identification algorithms demonstrated specificity >96%, whereas algorithm sensitivity was highest in the 29‐ to 56‐days‐old age group and lowest among infants 57 to 89 days old across all 4 algorithms (Figure 2). Similar to the overall cohort, an algorithm of discharge or admission diagnosis of fever demonstrated specificity of nearly 98% in all age groups; addition of serious infection codes to this algorithm increased sensitivity, highest in the 29‐ to 56‐days‐old age group (Figure 2; see also Supporting Information, Table 2, in the online version of this article).
ICD‐9 Diagnosis Code Algorithm | Sensitivity, Median % (Range) | Specificity, Median % (Range) | Negative Predictive Value, Median % (Range) | Positive Predictive Value, Median % (Range) |
---|---|---|---|---|
| ||||
Discharge diagnosis of fever | 56.2 (34.681.0) | 98.3 (96.499.1) | 92.1 (83.297.4) | 87.7 (74.093.2) |
Discharge or Admission diagnosis of Fever | 76.7 (51.385.0) | 97.8 (96.298.7) | 95.6 (86.997.4) | 87.4 (80.092.9) |
Discharge diagnosis of fever or serious infection | 68.3 (44.287.3) | 96.5 (95.498.0) | 93.6 (85.298.2) | 78.3 (74.289.0) |
Discharge or admission diagnosis of fever or serious infection | 83.1 (58.390.7) | 95.8 (95.498.0) | 96.5 (88.598.2) | 79.1 (77.490.4) |
Across the 8 study sites, median specificity was 95.8% to 98.3% for the 4 algorithms, with little interhospital variability; however, algorithm sensitivity varied widely by site. Median PPV was highest for discharge diagnosis of fever alone at 87.7% but ranged from 74.0% to 93.2% across sites. Median PPV for an algorithm of discharge or admission diagnosis of fever was similar (87.4%) but with less variation by site (range 80.0%92.9%) (Table 2).
Outcomes by ICD‐9 Diagnosis Code Group and Case‐Identification Algorithm
When compared with discharge diagnosis of fever, adding admission diagnosis of fever captured a higher proportion of hospitalized infants with SBIs (UTI/pyelonephritis, bacteremia/sepsis, or bacterial meningitis). However, median hospital length of stay, severe outcomes, and 3‐day revisits and revisits with hospitalization did not materially differ when including infants with admission diagnosis of fever in addition to discharge diagnosis of fever. Addition of infants with a diagnosis code for serious infection substantially increased the number of infants with SBIs and severe outcomes but did not capture additional 3‐day revisits (Table 3). There were no additional cases of SBI in the no fever/no serious illness diagnosis code group.
ICD‐9 Diagnosis Code Algorithm | Outcome | 3‐Day Revisit, % (95% CI) | 3‐Day Revisit With Hospitalization, % (95% CI) | |||
---|---|---|---|---|---|---|
Hospitalized, % (95% CI) | UTI/Pyelonephritis, Bacteremia/Sepsis, or Bacterial Meningitis, % (95% CI) | Severe Outcome, % (95% CI)* | Length of Stay in Days, Median (IQR) | |||
| ||||||
Discharge diagnosis of fever | 44.3 (40.348.4) | 3.3 (1.84.7) | 1.4 (0.42.3) | 3 (23) | 11.7 (8.215.2) | 5.9 (3.38.4) |
Discharge or admission diagnosis of fever | 52.4 (48.955.9) | 6.1 (4.47.8) | 1.9 (1.02.9) | 3 (23) | 10.9 (7.714.1) | 5.4 (3.17.8) |
Discharge diagnosis of fever or serious infection | 54.0 (50.457.5) | 15.3 (12.717.8) | 3.8 (2.55.2) | 3 (24) | 11.0 (7.714.2) | 5.5 (3.17.9) |
Discharge or admission diagnosis of fever or serious infection | 56.5 (53.259.7) | 12.9 (10.715.1) | 3.6 (2.44.8) | 3 (24) | 10.3 (7.313.3) | 5.2 (3.07.4) |
Among infants who met the reference standard for fever but did not have a discharge or admission diagnosis of fever (false negatives), 11.8% had a diagnosis of SBI. Overall, 43.2% of febrile infants (and 84.4% of hospitalized infants) with SBI did not have an ICD‐9 discharge or admission diagnosis of fever. Addition of ICD‐9 diagnosis codes of serious infection to the algorithm of discharge or admission diagnosis of fever captured all additional SBIs, and no false negativeinfants missed with this algorithm had an SBI.
DISCUSSION
We described the performance of 4 ICD‐9 diagnosis code case‐identification algorithms for the identification of febrile young infants <90 days of age at US children's hospitals. Although the specificity was high across algorithms and institutions, the sensitivity was relatively low, particularly for discharge diagnosis of fever, and varied by institution. Given the high specificity, ICD‐9 diagnosis code case‐identification algorithms for fever reliably identify febrile infants using administrative data with low rates of inclusion of infants without fever. However, underidentification of patients, particularly those more prone to SBIs and severe outcomes depending on the algorithm utilized, can impact interpretation of comparative effectiveness studies or the quality of care delivered by an institution.
ICD‐9 discharge diagnosis codes are frequently used to identify pediatric patients across a variety of administrative databases, diseases, and symptoms.[19, 27, 28, 29, 30, 31] Although discharge diagnosis of fever is highly specific, sensitivity is substantially lower than other case‐identification algorithms we studied, particularly for hospitalized infants. This may be due to a fever code sometimes being omitted in favor of a more specific diagnosis (eg, bacteremia) prior to hospital discharge. Therefore, case identification relying only on ICD‐9 discharge diagnosis codes for fever may under‐report clinically important SBI or severe outcomes as demonstrated in our study. This is in contrast to ICD‐9 diagnosis code identification strategies for childhood UTI and pneumonia, which largely have higher sensitivity but lower specificity than fever codes.[13, 14]
Admission diagnosis of fever is important for febrile infants as they may not have an explicit diagnosis at the time of disposition from the ED. Addition of admission diagnosis of fever to an algorithm relying on discharge diagnosis code alone increased sensitivity without a demonstrable reduction in specificity and PPV, likely due to capture of infants with a fever diagnosis at presentation before a specific infection was identified. Although using an algorithm of discharge or admission diagnosis of fever captured a higher percentage of hospitalized febrile infants with SBIs, sensitivity was only 71% overall with this algorithm, and 43% of febrile infants with SBI would still have been missed. Importantly, though, addition of various ICD‐9 codes for serious infection to this algorithm resulted in capture of all febrile infants with SBI and should be used as a sensitivity analysis.
The test characteristics of diagnosis codes were highest in the 29‐ to 56‐days‐old age group. Given the differing low‐risk criteria[6, 7, 8] and lack of best practice guidelines[16] in this age group, the use of administrative data may allow for the comparison of testing and treatment strategies across a large cohort of febrile infants aged 29 to 56 days. However, individual hospital coding practices may affect algorithm performance, in particular sensitivity, which varied substantially by hospital. This variation in algorithm sensitivity may impact comparisons of outcomes across institutions. Therefore, when conducting studies of febrile infants using administrative data, sensitivity analyses or use of chart review should be considered to augment the use of ICD‐9 code‐based identification strategies, particularly for comparative benchmarking and outcomes studies. These additional analyses are particularly important for studies of febrile infants >56 days of age, in whom the sensitivity of diagnosis codes is particularly low. We speculate that the lower sensitivity in older febrile infants may relate to a lack of consensus on the clinical significance of fever in this age group and the varying management strategies employed.[10]
Strengths of this study include the assessment of ICD‐9 code algorithms across multiple institutions for identification of fever in young infants, and the patterns of our findings remained robust when comparing median performance characteristics of the algorithms across hospitals to our overall findings. We were also able to accurately estimate PPV and NPV using a case‐identification strategy weighted to the actual population sizes. Although sensitivity and specificity are the primary measures of test performance, predictive values are highly informative for investigators using administrative data. Additionally, our findings may inform public health efforts including disease surveillance, assessment of seasonal variation, and identification and monitoring of healthcare‐associated infections among febrile infants.
Our study has limitations. We did not review all identified records, which raises the possibility that our evaluated cohort may not be representative of the entire febrile infant population. We attempted to mitigate this possibility by using a random sampling strategy for our population selection that was weighted to the actual population sizes. Second, we identified serious infections using ICD‐9 diagnosis codes determined by group consensus, which may not capture all serious infection codes that identify febrile infants whose fever code was omitted. Third, 47 infants had abnormal temperature that did not meet our reference standard criteria for fever and were included in the no fever group. Although there may be disagreement regarding what constitutes a fever, we used a widely accepted reference standard to define fever.[16] Further, inclusion of these 47 infants as fever did not materially change algorithm performance. Last, our study was conducted at 8 large tertiary‐care children's hospitals, and our results may not be generalizable to other children's hospitals and community‐based hospitals.
CONCLUSIONS
Studies of febrile young infants that rely on ICD‐9 discharge diagnosis code of fever for case ascertainment have high specificity but low sensitivity for the identification of febrile infants, particularly among hospitalized patients. A case‐identification strategy that includes discharge or admission diagnosis of fever demonstrated higher sensitivity, and should be considered for studies of febrile infants using administrative data. However, additional strategies such as incorporation of ICD‐9 codes for serious infection should be used when comparing outcomes across institutions.
Acknowledgements
The Febrile Young Infant Research Collaborative includes the following additional collaborators who are acknowledged for their work on this study: Erica DiLeo, MA, Department of Medical Education and Research, Danbury Hospital, Danbury, Connecticut; Janet Flores, BS, Division of Emergency Medicine, Ann and Robert H. Lurie Children's Hospital of Chicago, Chicago, Illinois.
Disclosures: This project funded in part by The Gerber Foundation Novice Researcher Award, (Ref No. 1827‐3835). Dr. Fran Balamuth received career development support from the National Institutes of Health (NHLBI K12‐HL109009). Funders were not involved in the design or conduct of the study; collection, management, analysis, or interpretation of the data; or preparation, review, or approval of the manuscript. The authors have no conflicts of interest relevant to this article to disclose.
- The prevalence of serious bacterial infections by age in febrile infants during the first 3 months of life. Pediatr Ann. 1993;22:462–466. .
- Performance of low‐risk criteria in the evaluation of young infants with fever: review of the literature. Pediatrics. 2010;125:228–233. , , .
- A week‐by‐week analysis of the low‐risk criteria for serious bacterial infection in febrile neonates. Arch Dis Child. 2009;94:287–292. , , , , , .
- Is 15 days an appropriate cut‐off age for considering serious bacterial infection in the management of febrile infants? Pediatr Infect Dis J. 2012;31:455–458. , , , et al.
- Failure of infant observation scales in detecting serious illness in febrile, 4‐ to 8‐week‐old infants. Pediatrics. 1990;85:1040–1043. , , .
- Outpatient management without antibiotics of fever in selected infants. N Engl J Med. 1993;329:1437–1441. , , .
- Identifying febrile infants at risk for a serious bacterial infection. J Pediatr. 1993;123:489–490. , , .
- Febrile infants at low risk for serious bacterial infection—an appraisal of the Rochester criteria and implications for management. Febrile Infant Collaborative Study Group. Pediatrics. 1994;94:390–396. , , , et al.
- Management of febrile neonates in US pediatric emergency departments. Pediatrics. 2014;133:187–195. , , , et al.
- Variation in care of the febrile young infant <90 days in US pediatric emergency departments. Pediatrics. 2014;134:667–677. , , , et al.
- Association of clinical practice guidelines with emergency department management of febrile infants ≤56 days of age. J Hosp Med. 2015;10:358–365. , , , et al.
- Diagnosis and management of febrile infants (0‐3 months). Evid Rep Technol Assess (Full Rep). 2012;(205):1–297. , , , et al.
- Accuracy of administrative billing codes to detect urinary tract infection hospitalizations. Pediatrics. 2011;128:323–330. , , , et al.
- Identifying pediatric community‐acquired pneumonia hospitalizations: accuracy of administrative billing codes. JAMA Pediatr. 2013;167:851–858. , , , et al.
- Development and use of reporting guidelines for assessing the quality of validation studies of health administrative data. J Clin Epidemiol. 2011;64:821–829. , , , , , .
- American College of Emergency Physicians Clinical Policies Committee; American College of Emergency Physicians Clinical Policies Subcommittee on Pediatric Fever. Clinical policy for children younger than three years presenting to the emergency department with fever. Ann Emerg Med. 2003;42:530–545.
- Variation in occult injury screening for children with suspected abuse in selected US children's hospitals. Pediatrics. 2012;130:853–860. , , , , , .
- Achieving data quality. How data from a pediatric health information system earns the trust of its users. J AHIMA. 2004;75:22–26. .
- Corticosteroids and mortality in children with bacterial meningitis. JAMA. 2008;299:2048–2055. , , , .
- Research electronic data capture (REDCap)—a metadata‐driven methodology and workflow process for providing translational research informatics support. J Biomed Inform. 2009;42:377–381. , , , , , .
- Deaths attributed to pediatric complex chronic conditions: national trends and implications for supportive care services. Pediatrics. 2001;107:E99. , , , , , .
- US Census Bureau. Geographic terms and concepts—census divisions and census regions. Available at: https://www.census.gov/geo/reference/gtc/gtc_census_divreg.html. Accessed October 20, 2014.
- Initial emergency department diagnosis and return visits: risk versus perception. Ann Emerg Med. 1998;32:569–573. , , , .
- A national depiction of children with return visits to the emergency department within 72 hours, 2001–2007. Pediatr Emerg Care. 2012;28:606–610. , , , , .
- Pediatric observation status: are we overlooking a growing population in children's hospitals? J Hosp Med. 2012;7:530–536. , , , et al.
- Differences in designations of observation care in US freestanding children's hospitals: are they virtual or real? J Hosp Med. 2012;7:287–293. , , , et al.
- Trends in the management of viral meningitis at United States children's hospitals. Pediatrics. 2013;131:670–676. , , , , .
- Impact of increasing ondansetron use on clinical outcomes in children with gastroenteritis. JAMA Pediatr. 2014;168:321–329. , , , et al.
- Race, otitis media, and antibiotic selection. Pediatrics. 2014;134:1059–1066. , , , , .
- Establishing benchmarks for the hospitalized care of children with asthma, bronchiolitis, and pneumonia. Pediatrics. 2014;134:555–562. , , , et al.
- Diagnostic testing and treatment of pediatric headache in the emergency department. J Pediatr. 2013;163:1634–1637. , , , , .
- The prevalence of serious bacterial infections by age in febrile infants during the first 3 months of life. Pediatr Ann. 1993;22:462–466. .
- Performance of low‐risk criteria in the evaluation of young infants with fever: review of the literature. Pediatrics. 2010;125:228–233. , , .
- A week‐by‐week analysis of the low‐risk criteria for serious bacterial infection in febrile neonates. Arch Dis Child. 2009;94:287–292. , , , , , .
- Is 15 days an appropriate cut‐off age for considering serious bacterial infection in the management of febrile infants? Pediatr Infect Dis J. 2012;31:455–458. , , , et al.
- Failure of infant observation scales in detecting serious illness in febrile, 4‐ to 8‐week‐old infants. Pediatrics. 1990;85:1040–1043. , , .
- Outpatient management without antibiotics of fever in selected infants. N Engl J Med. 1993;329:1437–1441. , , .
- Identifying febrile infants at risk for a serious bacterial infection. J Pediatr. 1993;123:489–490. , , .
- Febrile infants at low risk for serious bacterial infection—an appraisal of the Rochester criteria and implications for management. Febrile Infant Collaborative Study Group. Pediatrics. 1994;94:390–396. , , , et al.
- Management of febrile neonates in US pediatric emergency departments. Pediatrics. 2014;133:187–195. , , , et al.
- Variation in care of the febrile young infant <90 days in US pediatric emergency departments. Pediatrics. 2014;134:667–677. , , , et al.
- Association of clinical practice guidelines with emergency department management of febrile infants ≤56 days of age. J Hosp Med. 2015;10:358–365. , , , et al.
- Diagnosis and management of febrile infants (0‐3 months). Evid Rep Technol Assess (Full Rep). 2012;(205):1–297. , , , et al.
- Accuracy of administrative billing codes to detect urinary tract infection hospitalizations. Pediatrics. 2011;128:323–330. , , , et al.
- Identifying pediatric community‐acquired pneumonia hospitalizations: accuracy of administrative billing codes. JAMA Pediatr. 2013;167:851–858. , , , et al.
- Development and use of reporting guidelines for assessing the quality of validation studies of health administrative data. J Clin Epidemiol. 2011;64:821–829. , , , , , .
- American College of Emergency Physicians Clinical Policies Committee; American College of Emergency Physicians Clinical Policies Subcommittee on Pediatric Fever. Clinical policy for children younger than three years presenting to the emergency department with fever. Ann Emerg Med. 2003;42:530–545.
- Variation in occult injury screening for children with suspected abuse in selected US children's hospitals. Pediatrics. 2012;130:853–860. , , , , , .
- Achieving data quality. How data from a pediatric health information system earns the trust of its users. J AHIMA. 2004;75:22–26. .
- Corticosteroids and mortality in children with bacterial meningitis. JAMA. 2008;299:2048–2055. , , , .
- Research electronic data capture (REDCap)—a metadata‐driven methodology and workflow process for providing translational research informatics support. J Biomed Inform. 2009;42:377–381. , , , , , .
- Deaths attributed to pediatric complex chronic conditions: national trends and implications for supportive care services. Pediatrics. 2001;107:E99. , , , , , .
- US Census Bureau. Geographic terms and concepts—census divisions and census regions. Available at: https://www.census.gov/geo/reference/gtc/gtc_census_divreg.html. Accessed October 20, 2014.
- Initial emergency department diagnosis and return visits: risk versus perception. Ann Emerg Med. 1998;32:569–573. , , , .
- A national depiction of children with return visits to the emergency department within 72 hours, 2001–2007. Pediatr Emerg Care. 2012;28:606–610. , , , , .
- Pediatric observation status: are we overlooking a growing population in children's hospitals? J Hosp Med. 2012;7:530–536. , , , et al.
- Differences in designations of observation care in US freestanding children's hospitals: are they virtual or real? J Hosp Med. 2012;7:287–293. , , , et al.
- Trends in the management of viral meningitis at United States children's hospitals. Pediatrics. 2013;131:670–676. , , , , .
- Impact of increasing ondansetron use on clinical outcomes in children with gastroenteritis. JAMA Pediatr. 2014;168:321–329. , , , et al.
- Race, otitis media, and antibiotic selection. Pediatrics. 2014;134:1059–1066. , , , , .
- Establishing benchmarks for the hospitalized care of children with asthma, bronchiolitis, and pneumonia. Pediatrics. 2014;134:555–562. , , , et al.
- Diagnostic testing and treatment of pediatric headache in the emergency department. J Pediatr. 2013;163:1634–1637. , , , , .
© 2015 Society of Hospital Medicine
Febrile Infant CPGs
Febrile young infants are at high risk for serious bacterial infection (SBI) with reported rates of 8.5% to 12%, even higher in neonates 28 days of age.[1, 2, 3] As a result, febrile infants often undergo extensive diagnostic evaluation consisting of a combination of urine, blood, and cerebrospinal fluid (CSF) testing.[4, 5, 6] Several clinical prediction algorithms use this diagnostic testing to identify febrile infants at low risk for SBI, but they differ with respect to age range, recommended testing, antibiotic administration, and threshold for hospitalization.[4, 5, 6] Additionally, the optimal management strategy for this population has not been defined.[7] Consequently, laboratory testing, antibiotic use, and hospitalization for febrile young infants vary widely among hospitals.[8, 9, 10]
Clinical practice guidelines (CPGs) are designed to implement evidence‐based care and reduce practice variability, with the goal of improving quality of care and optimizing costs.[11] Implementation of a CPG for management of febrile young infants in the Intermountain Healthcare System was associated with greater adherence to evidence‐based care and lower costs.[12] However, when strong evidence is lacking, different interpretations of febrile infant risk classification incorporated into local CPGs may be a major driver of the across‐hospital practice variation observed in prior studies.[8, 9] Understanding sources of variability as well as determining the association of CPGs with clinicians' practice patterns can help identify quality improvement opportunities, either through national benchmarking or local efforts.
Our primary objectives were to compare (1) recommendations of pediatric emergency departmentbased institutional CPGs for febrile young infants and (2) rates of urine, blood, CSF testing, hospitalization, and ceftriaxone use at emergency department (ED) discharge based upon CPG presence and the specific CPG recommendations. Our secondary objectives were to describe the association of CPGs with healthcare costs and return visits for SBI.
METHODS
Study Design
We used the Pediatric Health Information System (PHIS) to identify febrile infants 56 days of age who presented to the ED between January 1, 2013 and December 31, 2013. We also surveyed ED providers at participating PHIS hospitals. Informed consent was obtained from survey respondents. The institutional review board at Boston Children's Hospital approved the study protocol.
Clinical Practice Guideline Survey
We sent an electronic survey to medical directors or division directors at 37 pediatric EDs to determine whether their ED utilized a CPG for the management of the febrile young infant in 2013. If no response was received after the second attempt, we queried ED fellowship directors or other ED attending physicians at nonresponding hospitals. Survey items included the presence of a febrile young infant CPG, and if present, the year of implementation, ages targeted, and CPG content. As applicable, respondents were asked to share their CPG and/or provide the specific CPG recommendations.
We collected and managed survey data using the Research Electronic Data Capture (REDCap) electronic data capture tools hosted at Boston Children's Hospital. REDCap is a secure, Web‐based application designed to support data capture for research studies.[13]
Data Source
The PHIS database contains administrative data from 44 US children's hospitals. These hospitals, affiliated with the Children's Hospital Association, represent 85% of freestanding US children's hospitals.[14] Encrypted patient identifiers permit tracking of patients across encounters.[15] Data quality and integrity are assured jointly by the Children's Hospital Association and participating hospitals.[16] For this study, 7 hospitals were excluded due to incomplete ED data or known data‐quality issues.[17]
Patients
We identified study infants using the following International Classification of Diseases, 9th Revision (ICD‐9) admission or discharge diagnosis codes for fever as defined previously[8, 9]: 780.6, 778.4, 780.60, or 780.61. We excluded infants with a complex chronic condition[18] and those transferred from another institution, as these infants may warrant a nonstandard evaluation and/or may have incomplete data. For infants with >1 ED visit for fever during the study period, repeat visits within 3 days of an index visit were considered a revisit for the same episode of illness; visits >3 days following an index visit were considered as a new index visit.
Study Definitions
From the PHIS database, we abstracted demographic characteristics (gender, race/ethnicity), insurance status, and region where the hospital was located (using US Census categories[19]). Billing codes were used to assess whether urine, blood, and CSF testing (as defined previously[9]) were performed during the ED evaluation. To account for ED visits that spanned the midnight hour, for hospitalized patients we considered any testing or treatment occurring on the initial or second hospital day to be performed in the ED; billing code data in PHIS are based upon calendar day and do not distinguish testing performed in the ED versus inpatient setting.[8, 9] Patients billed for observation care were classified as being hospitalized.[20, 21]
We identified the presence of an SBI using ICD‐9 diagnosis codes for the following infections as described previously[9]: urinary tract infection or pyelonephritis,[22] bacteremia or sepsis, bacterial meningitis,[16] pneumonia,[23] or bacterial enteritis. To assess return visits for SBI that required inpatient management, we defined an ED revisit for an SBI as a return visit within 3 days of ED discharge[24, 25] that resulted in hospitalization with an associated ICD‐9 discharge diagnosis code for an SBI.
Hospitals charges in PHIS database were adjusted for hospital location by using the Centers for Medicare and Medicaid Services price/wage index. Costs were estimated by applying hospital‐level cost‐to‐charge ratios to charge data.[26]
Measured Exposures
The primary exposure was the presence of an ED‐based CPG for management of the febrile young infant aged 28 days and 29 to 56 days; 56 days was used as the upper age limit as all of the CPGs included infants up to this age or beyond. Six institutions utilized CPGs with different thresholds to define the age categories (eg, dichotomized at 27 or 30 days); these CPGs were classified into the aforementioned age groups to permit comparisons across standardized age groups. We classified institutions based on the presence of a CPG. To assess differences in the application of low‐risk criteria, the CPGs were further classified a priori based upon specific recommendations around laboratory testing and hospitalization, as well as ceftriaxone use for infants aged 29 to 56 days discharged from the ED. CPGs were categorized based upon whether testing, hospitalization, and ceftriaxone use were: (1) recommended for all patients, (2) recommended only if patients were classified as high risk (absence of low‐risk criteria), (3) recommended against, or (4) recommended to consider at clinician discretion.
Outcome Measures
Measured outcomes were performance of urine, blood, CSF testing, and hospitalization rate, as well as rate of ceftriaxone use for discharged infants aged 29 to 56 days, 3‐day revisits for SBI, and costs per visit, which included hospitalization costs for admitted patients.
Data Analysis
We described continuous variables using median and interquartile range or range values and categorical variables using frequencies. We compared medians using Wilcoxon rank sum and categorical variables using a [2] test. We compared rates of testing, hospitalization, ceftriaxone use, and 3‐day revisits for SBI based on the presence of a CPG, and when present, the specific CPG recommendations. Costs per visit were compared between institutions with and without CPGs and assessed separately for admitted and discharged patients. To adjust for potential confounders and clustering of patients within hospitals, we used generalized estimating equations with logistic regression to generate adjusted odd ratios (aORs) and 95% confidence intervals (CIs). Models were adjusted for geographic region, payer, race, and gender. Statistical analyses were performed by using SAS version 9.3 (SAS Institute, Cary, NC). We determined statistical significance as a 2‐tailed P value <0.05.
Febrile infants with bronchiolitis or a history of prematurity may be managed differently from full‐term febrile young infants without bronchiolitis.[6, 27] Therefore, we performed a subgroup analysis after exclusion of infants with an ICD‐9 discharge diagnosis code for bronchiolitis (466.11 and 466.19)[28] or prematurity (765).
Because our study included ED encounters in 2013, we repeated our analyses after exclusion of hospitals with CPGs implemented during the 2013 calendar year.
RESULTS
CPG by Institution
Thirty‐three (89.2%) of the 37 EDs surveyed completed the questionnaire. Overall, 21 (63.6%) of the 33 EDs had a CPG; 15 (45.5%) had a CPG for all infants 56 days of age, 5 (15.2%) had a CPG for infants 28 days only, and 1 (3.0%) had a CPG for infants 29 to 56 days but not 28 days of age (Figure 1). Seventeen EDs had an established CPG prior to 2013, and 4 hospitals implemented a CPG during the 2013 calendar year, 2 with CPGs for neonates 28 days and 2 with CPGs for both 28 days and 29 to 56 days of age. Hospitals with CPGs were more likely to be located in the Northeast and West regions of the United States and provide care to a higher proportion of non‐Hispanic white patients, as well as those with commercial insurance (Table 1).
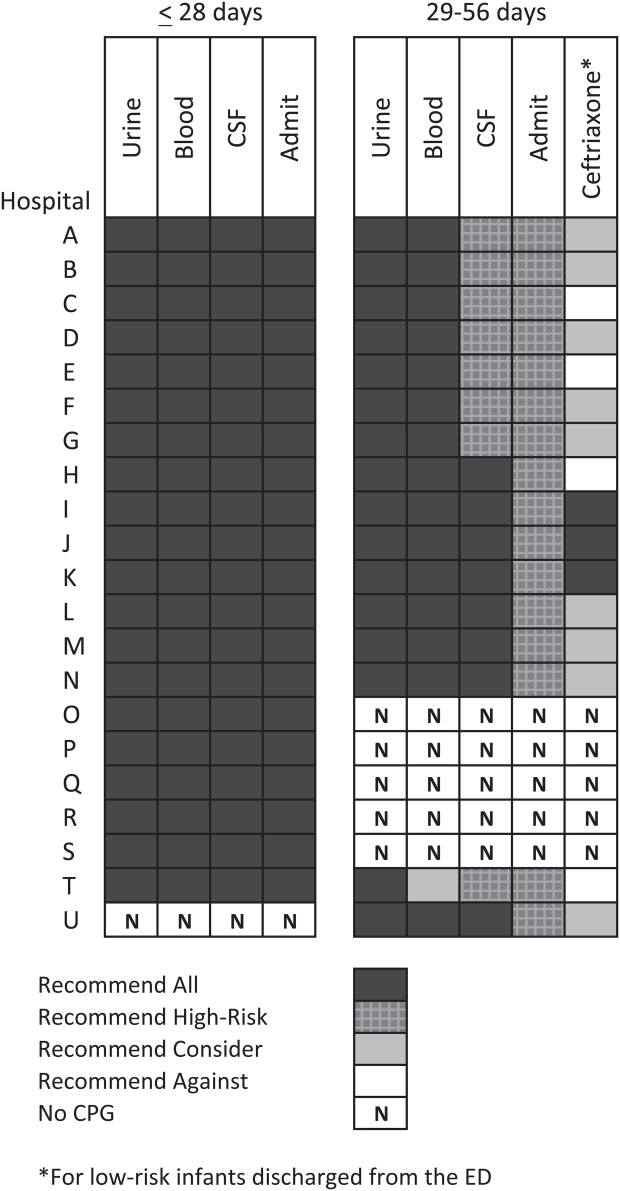
Characteristic | 28 Days | 2956 Days | ||||
---|---|---|---|---|---|---|
No CPG, n=996, N (%) | CPG, n=2,149, N (%) | P Value | No CPG, n=2,460, N (%) | CPG, n=3,772, N (%) | P Value | |
| ||||||
Race | ||||||
Non‐Hispanic white | 325 (32.6) | 996 (46.3) | 867 (35.2) | 1,728 (45.8) | ||
Non‐Hispanic black | 248 (24.9) | 381 (17.7) | 593 (24.1) | 670 (17.8) | ||
Hispanic | 243 (24.4) | 531 (24.7) | 655 (26.6) | 986 (26.1) | ||
Asian | 28 (2.8) | 78 (3.6) | 40 (1.6) | 122 (3.2) | ||
Other Race | 152 (15.3) | 163 (7.6) | <0.001 | 305 (12.4) | 266 (7.1) | <0.001 |
Gender | ||||||
Female | 435 (43.7) | 926 (43.1) | 0.76 | 1,067 (43.4) | 1,714 (45.4) | 0.22 |
Payer | ||||||
Commercial | 243 (24.4) | 738 (34.3) | 554 (22.5) | 1,202 (31.9) | ||
Government | 664 (66.7) | 1,269 (59.1) | 1,798 (73.1) | 2,342 (62.1) | ||
Other payer | 89 (8.9) | 142 (6.6) | <0.001 | 108 (4.4) | 228 (6.0) | <0.001 |
Region | ||||||
Northeast | 39 (3.9) | 245 (11.4) | 77 (3.1) | 572 (15.2) | ||
South | 648 (65.1) | 915 (42.6) | 1,662 (67.6) | 1,462 (38.8) | ||
Midwest | 271 (27.2) | 462 (21.5) | 506 (20.6) | 851 (22.6) | ||
West | 38 (3.8) | 527 (24.5) | <0.001 | 215 (8.7) | 887 (23.5) | <0.001 |
Serious bacterial infection | ||||||
Overall* | 131 (13.2) | 242 (11.3) | 0.14 | 191 (7.8) | 237 (6.3) | 0.03 |
UTI/pyelonephritis | 73 (7.3) | 153 (7.1) | 103 (4.2) | 154 (4.1) | ||
Bacteremia/sepsis | 56 (5.6) | 91 (4.2) | 78 (3.2) | 61 (1.6) | ||
Bacterial meningitis | 15 (1.5) | 15 (0.7) | 4 (0.2) | 14 (0.4) | ||
Age, d, median (IQR) | 18 (11, 24) | 18 (11, 23) | 0.67 | 46 (37, 53) | 45 (37, 53) | 0.11 |
All 20 CPGs for the febrile young infant 28 days of age recommended urine, blood, CSF testing, and hospitalization for all infants (Figure 1). Of the 16 hospitals with CPGs for febrile infants aged 29 to 56 days, all recommended urine and blood testing for all patients, except for 1 CPG, which recommended consideration of blood testing but not to obtain routinely. Hospitals varied in recommendations for CSF testing among infants aged 29 to 56 days: 8 (50%) recommended CSF testing in all patients and 8 (50%) recommended CSF testing only if the patient was high risk per defined criteria (based on history, physical examination, urine, and blood testing). In all 16 CPGs, hospitalization was recommended only for high‐risk infants. For low‐risk infants aged 2956 days being discharged from the ED, 3 hospitals recommended ceftriaxone for all, 9 recommended consideration of ceftriaxone, and 4 recommended against antibiotics (Figure 1).
Study Patients
During the study period, there were 10,415 infants 56 days old with a diagnosis of fever at the 33 participating hospitals. After exclusion of 635 (6.1%) infants with a complex chronic condition and 445 (4.3%) transferred from another institution (including 42 with a complex chronic condition), 9377 infants remained in our study cohort. Approximately one‐third of the cohort was 28 days of age and two‐thirds aged 29 to 56 days. The overall SBI rate was 8.5% but varied by age (11.9% in infants 28 days and 6.9% in infants 29 to 56 days of age) (Table 1).
CPGs and Use of Diagnostic Testing, Hospitalization Rates, Ceftriaxone Use, and Revisits for SBI
For infants 28 days of age, the presence of a CPG was not associated with urine, blood, CSF testing, or hospitalization after multivariable adjustment (Table 2). Among infants aged 29 to 56 days, urine testing did not differ based on the presence of a CPG, whereas blood testing was performed less often at the 1 hospital whose CPG recommended to consider, but not routinely obtain, testing (aOR: 0.4, 95% CI: 0.3‐0.7, P=0.001). Compared to hospitals without a CPG, CSF testing was performed less often at hospitals with CPG recommendations to only obtain CSF if high risk (aOR: 0.5, 95% CI: 0.3‐0.8, P=0.002). However, the odds of hospitalization did not differ at institutions with and without a febrile infant CPG (aOR: 0.7, 95% CI: 0.5‐1.1, P=0.10). For infants aged 29 to 56 days discharged from the ED, ceftriaxone was administered more often at hospitals with CPGs that recommended ceftriaxone for all discharged patients (aOR: 4.6, 95% CI: 2.39.3, P<0.001) and less often at hospitals whose CPGs recommended against antibiotics (aOR: 0.3, 95% CI: 0.1‐0.9, P=0.03) (Table 3). Our findings were similar in the subgroup of infants without bronchiolitis or prematurity (see Supporting Tables 1 and 2 in the online version of this article). After exclusion of hospitals with a CPG implemented during the 2013 calendar year (4 hospitals excluded in the 28 days age group and 2 hospitals excluded in the 29 to 56 days age group), infants aged 29 to 56 days cared for at a hospital with a CPG experienced a lower odds of hospitalization (aOR: 0.7, 95% CI: 0.4‐0.98, P=0.04). Otherwise, our findings in both age groups did not materially differ from the main analyses.
Testing/Hospitalization | No. of Hospitals | No. of Patients | % Received* | aOR (95% CI) | P Value |
---|---|---|---|---|---|
| |||||
Laboratory testing | |||||
Urine testing | |||||
No CPG | 13 | 996 | 75.6 | Ref | |
CPG: recommend for all | 20 | 2,149 | 80.7 | 1.2 (0.9‐1.7) | 0.22 |
Blood testing | |||||
No CPG | 13 | 996 | 76.9 | Ref | |
CPG: recommend for all | 20 | 2,149 | 81.8 | 1.2 (0.9‐1.7) | 0.25 |
CSF testing‖ | |||||
No CPG | 13 | 996 | 71.0 | Ref | |
CPG: recommend for all | 20 | 2,149 | 77.5 | 1.3 (1.01.7) | 0.08 |
Disposition | |||||
Hospitalization | |||||
No CPG | 13 | 996 | 75.4 | Ref | |
CPG: recommend for all | 20 | 2,149 | 81.6 | 1.2 (0.9‐1.8) | 0.26 |
Testing/Hospitalization | No. of Hospitals | No. of Patients | % Received* | aOR (95% CI) | P Value |
---|---|---|---|---|---|
| |||||
Laboratory resting | |||||
Urine testing | |||||
No CPG | 17 | 2,460 | 81.1 | Ref | |
CPG: recommend for all | 16 | 3,772 | 82.1 | 0.9 (0.7‐1.4) | 0.76 |
Blood testing | |||||
No CPG | 17 | 2,460 | 79.4 | Ref | |
CPG: recommend for all | 15 | 3,628 | 82.6 | 1.1 (0.7‐1.6) | 0.70 |
CPG: recommend consider | 1 | 144 | 62.5 | 0.4 (0.3‐0.7) | 0.001 |
CSF testing‖ | |||||
No CPG | 17 | 2,460 | 46.3 | Ref | |
CPG: recommend for all | 8 | 1,517 | 70.3 | 1.3 (0.9‐1.9) | 0.11 |
CPG: recommend if high‐risk | 8 | 2,255 | 39.9 | 0.5 (0.3‐0.8) | 0.002 |
Disposition | |||||
Hospitalization | |||||
No CPG | 17 | 2,460 | 47.0 | Ref | |
CPG: recommend if high‐risk | 16 | 3,772 | 42.0 | 0.7 (0.5‐1.1) | 0.10 |
Ceftriaxone if discharged | |||||
No CPG | 17 | 1,304 | 11.7 | Ref | |
CPG: recommend against | 4 | 313 | 10.9 | 0.3 (0.1‐0.9) | 0.03 |
CPG: recommend consider | 9 | 1,567 | 14.4 | 1.5 (0.9‐2.4) | 0.09 |
CPG: recommend for all | 3 | 306 | 64.1 | 4.6 (2.39.3) | < 0.001 |
Three‐day revisits for SBI were similarly low at hospitals with and without CPGs among infants 28 days (1.5% vs 0.8%, P=0.44) and 29 to 56 days of age (1.4% vs 1.1%, P=0.44) and did not differ after exclusion of hospitals with a CPG implemented in 2013.
CPGs and Costs
Among infants 28 days of age, costs per visit did not differ for admitted and discharged patients based on CPG presence. The presence of an ED febrile infant CPG was associated with higher costs for both admitted and discharged infants 29 to 56 days of age (Table 4). The cost analysis did not significantly differ after exclusion of hospitals with CPGs implemented in 2013.
28 Days, Cost, Median (IQR) | 29 to 56 Days, Cost, Median (IQR) | |||||
---|---|---|---|---|---|---|
No CPG | CPG | P Value | No CPG | CPG | P Value | |
| ||||||
Admitted | $4,979 ($3,408$6,607) [n=751] | $4,715 ($3,472$6,526) [n=1,753] | 0.79 | $3,756 ($2,725$5,041) [n=1,156] | $3,923 ($3,077$5,243) [n=1,586] | <0.001 |
Discharged | $298 ($166$510) [n=245] | $231 ($160$464) [n=396] | 0.10 | $681($398$982) [n=1,304)] | $764 ($412$1,100) [n=2,186] | <0.001 |
DISCUSSION
We described the content and association of CPGs with management of the febrile infant 56 days of age across a large sample of children's hospitals. Nearly two‐thirds of included pediatric EDs have a CPG for the management of young febrile infants. Management of febrile infants 28 days was uniform, with a majority hospitalized after urine, blood, and CSF testing regardless of the presence of a CPG. In contrast, CPGs for infants 29 to 56 days of age varied in their recommendations for CSF testing as well as ceftriaxone use for infants discharged from the ED. Consequently, we observed considerable hospital variability in CSF testing and ceftriaxone use for discharged infants, which correlates with variation in the presence and content of CPGs. Institutional CPGs may be a source of the across‐hospital variation in care of febrile young infants observed in prior study.[9]
Febrile infants 28 days of age are at particularly high risk for SBI, with a prevalence of nearly 20% or higher.[2, 3, 29] The high prevalence of SBI, combined with the inherent difficulty in distinguishing neonates with and without SBI,[2, 30] has resulted in uniform CPG recommendations to perform the full‐sepsis workup in this young age group. Similar to prior studies,[8, 9] we observed that most febrile infants 28 days undergo the full sepsis evaluation, including CSF testing, and are hospitalized regardless of the presence of a CPG.
However, given the conflicting recommendations for febrile infants 29 to 56 days of age,[4, 5, 6] the optimal management strategy is less certain.[7] The Rochester, Philadelphia, and Boston criteria, 3 published models to identify infants at low risk for SBI, primarily differ in their recommendations for CSF testing and ceftriaxone use in this age group.[4, 5, 6] Half of the CPGs recommended CSF testing for all febrile infants, and half recommended CSF testing only if the infant was high risk. Institutional guidelines that recommended selective CSF testing for febrile infants aged 29 to 56 days were associated with lower rates of CSF testing. Furthermore, ceftriaxone use varied based on CPG recommendations for low‐risk infants discharged from the ED. Therefore, the influence of febrile infant CPGs mainly relates to the limiting of CSF testing and targeted ceftriaxone use in low‐risk infants. As the rate of return visits for SBI is low across hospitals, future study should assess outcomes at hospitals with CPGs recommending selective CSF testing. Of note, infants 29 to 56 days of age were less likely to be hospitalized when cared for at a hospital with an established CPG prior to 2013 without increase in 3‐day revisits for SBI. This finding may indicate that longer duration of CPG implementation is associated with lower rates of hospitalization for low‐risk infants; this finding merits further study.
The presence of a CPG was not associated with lower costs for febrile infants in either age group. Although individual healthcare systems have achieved lower costs with CPG implementation,[12] the mere presence of a CPG is not associated with lower costs when assessed across institutions. Higher costs for admitted and discharged infants 29 to 56 days of age in the presence of a CPG likely reflects the higher rate of CSF testing at hospitals whose CPGs recommend testing for all febrile infants, as well as inpatient management strategies for hospitalized infants not captured in our study. Future investigation should include an assessment of the cost‐effectiveness of the various testing and treatment strategies employed for the febrile young infant.
Our study has several limitations. First, the validity of ICD‐9 diagnosis codes for identifying young infants with fever is not well established, and thus our study is subject to misclassification bias. To minimize missed patients, we included infants with either an ICD‐9 admission or discharge diagnosis of fever; however, utilization of diagnosis codes for patient identification may have resulted in undercapture of infants with a measured temperature of 38.0C. It is also possible that some patients who did not undergo testing were misclassified as having a fever or had temperatures below standard thresholds to prompt diagnostic testing. This is a potential reason that testing was not performed in 100% of infants, even at hospitals with CPGs that recommended testing for all patients. Additionally, some febrile infants diagnosed with SBI may not have an associated ICD‐9 diagnosis code for fever. Although the overall SBI rate observed in our study was similar to prior studies,[4, 31] the rate in neonates 28 days of age was lower than reported in recent investigations,[2, 3] which may indicate inclusion of a higher proportion of low‐risk febrile infants. With the exception of bronchiolitis, we also did not assess diagnostic testing in the presence of other identified sources of infection such as herpes simplex virus.
Second, we were unable to assess the presence or absence of a CPG at the 4 excluded EDs that did not respond to the survey or the institutions excluded for data‐quality issues. However, included and excluded hospitals did not differ in region or annual ED volume (data not shown).
Third, although we classified hospitals based upon the presence and content of CPGs, we were unable to fully evaluate adherence to the CPG at each site.
Last, though PHIS hospitals represent 85% of freestanding children's hospitals, many febrile infants are hospitalized at non‐PHIS institutions; our results may not be generalizable to care provided at nonchildren's hospitals.
CONCLUSIONS
Management of febrile neonates 28 days of age does not vary based on CPG presence. However, CPGs for the febrile infant aged 29 to 56 days vary in recommendations for CSF testing as well as ceftriaxone use for low‐risk patients, which significantly contributes to practice variation and healthcare costs across institutions.
Acknowledgements
The Febrile Young Infant Research Collaborative includes the following additional investigators who are acknowledged for their work on this study: Kao‐Ping Chua, MD, Harvard PhD Program in Health Policy, Harvard University, Cambridge, Massachusetts, and Division of Emergency Medicine, Department of Pediatrics, Boston Children's Hospital, Boston, Massachusetts; Elana A. Feldman, BA, University of Washington School of Medicine, Seattle, Washington; and Katie L. Hayes, BS, Division of Emergency Medicine, Department of Pediatrics, The Children's Hospital of Philadelphia, Philadelphia, Pennsylvania.
Disclosures
This project was funded in part by The Gerber Foundation Novice Researcher Award (Ref #18273835). Dr. Fran Balamuth received career development support from the National Institutes of Health (NHLBI K12‐HL109009). Funders were not involved in design or conduct of the study; collection, management, analysis, or interpretation of the data; preparation, review, or approval of the manuscript. The authors have no financial relationships relevant to this article to disclose. No payment was received for the production of this article. The authors have no conflicts of interest relevant to this article to disclose.
- Performance of low‐risk criteria in the evaluation of young infants with fever: review of the literature. Pediatrics. 2010;125:228–233. , , .
- A week‐by‐week analysis of the low‐risk criteria for serious bacterial infection in febrile neonates. Arch Dis Child. 2009;94:287–292. , , , , , .
- Is 15 days an appropriate cut‐off age for considering serious bacterial infection in the management of febrile infants? Pediatr Infect Dis J. 2012;31:455–458. , , , et al.
- Outpatient management without antibiotics of fever in selected infants. N Engl J Med. 1993;329:1437–1441. , , .
- Identifying febrile infants at risk for a serious bacterial infection. J Pediatr. 1993;123:489–490. , , .
- Febrile infants at low risk for serious bacterial infection—an appraisal of the Rochester criteria and implications for management. Febrile Infant Collaborative Study Group. Pediatrics. 1994;94:390–396. , , , et al.
- American College of Emergency Physicians Clinical Policies Committee; American College of Emergency Physicians Clinical Policies Subcommittee on Pediatric Fever. Clinical policy for children younger than three years presenting to the emergency department with fever. Ann Emerg Med. 2003;42:530–545.
- Management of febrile neonates in US pediatric emergency departments. Pediatrics. 2014;133:187–195. , , , et al.
- Variation in care of the febrile young infant <90 days in US pediatric emergency departments. Pediatrics. 2014;134:667–677. , , , et al.
- Fever survey highlights significant variations in how infants aged ≤60 days are evaluated and underline the need for guidelines. Acta Paediatr. 2014;103:379–385. , , , , .
- Evidence‐based guidelines and critical pathways for quality improvement. Pediatrics. 1999;103:225–232. .
- Costs and infant outcomes after implementation of a care process model for febrile infants. Pediatrics. 2012;130:e16–e24. , , , et al.
- Research electronic data capture (REDCap)—a metadata‐driven methodology and workflow process for providing translational research informatics support. J Biomed Inform. 2009;42:377–381. , , , , , .
- Variation in occult injury screening for children with suspected abuse in selected US children's hospitals. Pediatrics. 2012;130:853–860. , , , , , .
- Achieving data quality. How data from a pediatric health information system earns the trust of its users. J AHIMA. 2004;75:22–26. .
- Corticosteroids and mortality in children with bacterial meningitis. JAMA. 2008;299:2048–2055. , , , .
- Variation in resource utilization across a national sample of pediatric emergency departments. J Pediatr. 2013;163:230–236. , , , et al.
- Deaths attributed to pediatric complex chronic conditions: national trends and implications for supportive care services. Pediatrics. 2001;107:E99. , , , , , .
- US Census Bureau. Geographic terms and concepts—census divisions and census regions. Available at: https://www.census.gov/geo/reference/gtc/gtc_census_divreg.html. Accessed September 10, 2014.
- Pediatric observation status: are we overlooking a growing population in children's hospitals? J Hosp Med. 2012;7:530–536. , , , et al.
- Differences in designations of observation care in US freestanding children's hospitals: are they virtual or real? J Hosp Med. 2012;7:287–293. , , , et al.
- Accuracy of administrative billing codes to detect urinary tract infection hospitalizations. Pediatrics. 2011;128:323–330. , , , et al.
- Identifying pediatric community‐acquired pneumonia hospitalizations: accuracy of administrative billing codes. JAMA Pediatr. 2013;167:851–858. , , , et al.
- Initial emergency department diagnosis and return visits: risk versus perception. Ann Emerg Med. 1998;32:569–573. , , , .
- A national depiction of children with return visits to the emergency department within 72 hours, 2001–2007. Pediatr Emerg Care. 2012;28:606–610. , , , , .
- Healthcare Cost and Utilization Project. Cost‐to‐charge ratio files. Available at: http://www.hcup‐us.ahrq.gov/db/state/costtocharge.jsp. Accessed September 11, 2014.
- Risk of serious bacterial infection in young febrile infants with respiratory syncytial virus infections. Pediatrics. 2004;113:1728–1734. , , , et al.
- Establishing benchmarks for the hospitalized care of children with asthma, bronchiolitis, and pneumonia. Pediatrics. 2014;134:555–562. , , , et al.
- Well appearing young infants with fever without known source in the emergency department: are lumbar punctures always necessary? Eur J Emerg Med. 2010;17:167–169. , , , , , .
- Unpredictability of serious bacterial illness in febrile infants from birth to 1 month of age. Arch Pediatr Adolesc Med. 1999;153:508–511. , .
- Management and outcomes of care of fever in early infancy. JAMA. 2004;291:1203–1212. , , , et al.
Febrile young infants are at high risk for serious bacterial infection (SBI) with reported rates of 8.5% to 12%, even higher in neonates 28 days of age.[1, 2, 3] As a result, febrile infants often undergo extensive diagnostic evaluation consisting of a combination of urine, blood, and cerebrospinal fluid (CSF) testing.[4, 5, 6] Several clinical prediction algorithms use this diagnostic testing to identify febrile infants at low risk for SBI, but they differ with respect to age range, recommended testing, antibiotic administration, and threshold for hospitalization.[4, 5, 6] Additionally, the optimal management strategy for this population has not been defined.[7] Consequently, laboratory testing, antibiotic use, and hospitalization for febrile young infants vary widely among hospitals.[8, 9, 10]
Clinical practice guidelines (CPGs) are designed to implement evidence‐based care and reduce practice variability, with the goal of improving quality of care and optimizing costs.[11] Implementation of a CPG for management of febrile young infants in the Intermountain Healthcare System was associated with greater adherence to evidence‐based care and lower costs.[12] However, when strong evidence is lacking, different interpretations of febrile infant risk classification incorporated into local CPGs may be a major driver of the across‐hospital practice variation observed in prior studies.[8, 9] Understanding sources of variability as well as determining the association of CPGs with clinicians' practice patterns can help identify quality improvement opportunities, either through national benchmarking or local efforts.
Our primary objectives were to compare (1) recommendations of pediatric emergency departmentbased institutional CPGs for febrile young infants and (2) rates of urine, blood, CSF testing, hospitalization, and ceftriaxone use at emergency department (ED) discharge based upon CPG presence and the specific CPG recommendations. Our secondary objectives were to describe the association of CPGs with healthcare costs and return visits for SBI.
METHODS
Study Design
We used the Pediatric Health Information System (PHIS) to identify febrile infants 56 days of age who presented to the ED between January 1, 2013 and December 31, 2013. We also surveyed ED providers at participating PHIS hospitals. Informed consent was obtained from survey respondents. The institutional review board at Boston Children's Hospital approved the study protocol.
Clinical Practice Guideline Survey
We sent an electronic survey to medical directors or division directors at 37 pediatric EDs to determine whether their ED utilized a CPG for the management of the febrile young infant in 2013. If no response was received after the second attempt, we queried ED fellowship directors or other ED attending physicians at nonresponding hospitals. Survey items included the presence of a febrile young infant CPG, and if present, the year of implementation, ages targeted, and CPG content. As applicable, respondents were asked to share their CPG and/or provide the specific CPG recommendations.
We collected and managed survey data using the Research Electronic Data Capture (REDCap) electronic data capture tools hosted at Boston Children's Hospital. REDCap is a secure, Web‐based application designed to support data capture for research studies.[13]
Data Source
The PHIS database contains administrative data from 44 US children's hospitals. These hospitals, affiliated with the Children's Hospital Association, represent 85% of freestanding US children's hospitals.[14] Encrypted patient identifiers permit tracking of patients across encounters.[15] Data quality and integrity are assured jointly by the Children's Hospital Association and participating hospitals.[16] For this study, 7 hospitals were excluded due to incomplete ED data or known data‐quality issues.[17]
Patients
We identified study infants using the following International Classification of Diseases, 9th Revision (ICD‐9) admission or discharge diagnosis codes for fever as defined previously[8, 9]: 780.6, 778.4, 780.60, or 780.61. We excluded infants with a complex chronic condition[18] and those transferred from another institution, as these infants may warrant a nonstandard evaluation and/or may have incomplete data. For infants with >1 ED visit for fever during the study period, repeat visits within 3 days of an index visit were considered a revisit for the same episode of illness; visits >3 days following an index visit were considered as a new index visit.
Study Definitions
From the PHIS database, we abstracted demographic characteristics (gender, race/ethnicity), insurance status, and region where the hospital was located (using US Census categories[19]). Billing codes were used to assess whether urine, blood, and CSF testing (as defined previously[9]) were performed during the ED evaluation. To account for ED visits that spanned the midnight hour, for hospitalized patients we considered any testing or treatment occurring on the initial or second hospital day to be performed in the ED; billing code data in PHIS are based upon calendar day and do not distinguish testing performed in the ED versus inpatient setting.[8, 9] Patients billed for observation care were classified as being hospitalized.[20, 21]
We identified the presence of an SBI using ICD‐9 diagnosis codes for the following infections as described previously[9]: urinary tract infection or pyelonephritis,[22] bacteremia or sepsis, bacterial meningitis,[16] pneumonia,[23] or bacterial enteritis. To assess return visits for SBI that required inpatient management, we defined an ED revisit for an SBI as a return visit within 3 days of ED discharge[24, 25] that resulted in hospitalization with an associated ICD‐9 discharge diagnosis code for an SBI.
Hospitals charges in PHIS database were adjusted for hospital location by using the Centers for Medicare and Medicaid Services price/wage index. Costs were estimated by applying hospital‐level cost‐to‐charge ratios to charge data.[26]
Measured Exposures
The primary exposure was the presence of an ED‐based CPG for management of the febrile young infant aged 28 days and 29 to 56 days; 56 days was used as the upper age limit as all of the CPGs included infants up to this age or beyond. Six institutions utilized CPGs with different thresholds to define the age categories (eg, dichotomized at 27 or 30 days); these CPGs were classified into the aforementioned age groups to permit comparisons across standardized age groups. We classified institutions based on the presence of a CPG. To assess differences in the application of low‐risk criteria, the CPGs were further classified a priori based upon specific recommendations around laboratory testing and hospitalization, as well as ceftriaxone use for infants aged 29 to 56 days discharged from the ED. CPGs were categorized based upon whether testing, hospitalization, and ceftriaxone use were: (1) recommended for all patients, (2) recommended only if patients were classified as high risk (absence of low‐risk criteria), (3) recommended against, or (4) recommended to consider at clinician discretion.
Outcome Measures
Measured outcomes were performance of urine, blood, CSF testing, and hospitalization rate, as well as rate of ceftriaxone use for discharged infants aged 29 to 56 days, 3‐day revisits for SBI, and costs per visit, which included hospitalization costs for admitted patients.
Data Analysis
We described continuous variables using median and interquartile range or range values and categorical variables using frequencies. We compared medians using Wilcoxon rank sum and categorical variables using a [2] test. We compared rates of testing, hospitalization, ceftriaxone use, and 3‐day revisits for SBI based on the presence of a CPG, and when present, the specific CPG recommendations. Costs per visit were compared between institutions with and without CPGs and assessed separately for admitted and discharged patients. To adjust for potential confounders and clustering of patients within hospitals, we used generalized estimating equations with logistic regression to generate adjusted odd ratios (aORs) and 95% confidence intervals (CIs). Models were adjusted for geographic region, payer, race, and gender. Statistical analyses were performed by using SAS version 9.3 (SAS Institute, Cary, NC). We determined statistical significance as a 2‐tailed P value <0.05.
Febrile infants with bronchiolitis or a history of prematurity may be managed differently from full‐term febrile young infants without bronchiolitis.[6, 27] Therefore, we performed a subgroup analysis after exclusion of infants with an ICD‐9 discharge diagnosis code for bronchiolitis (466.11 and 466.19)[28] or prematurity (765).
Because our study included ED encounters in 2013, we repeated our analyses after exclusion of hospitals with CPGs implemented during the 2013 calendar year.
RESULTS
CPG by Institution
Thirty‐three (89.2%) of the 37 EDs surveyed completed the questionnaire. Overall, 21 (63.6%) of the 33 EDs had a CPG; 15 (45.5%) had a CPG for all infants 56 days of age, 5 (15.2%) had a CPG for infants 28 days only, and 1 (3.0%) had a CPG for infants 29 to 56 days but not 28 days of age (Figure 1). Seventeen EDs had an established CPG prior to 2013, and 4 hospitals implemented a CPG during the 2013 calendar year, 2 with CPGs for neonates 28 days and 2 with CPGs for both 28 days and 29 to 56 days of age. Hospitals with CPGs were more likely to be located in the Northeast and West regions of the United States and provide care to a higher proportion of non‐Hispanic white patients, as well as those with commercial insurance (Table 1).
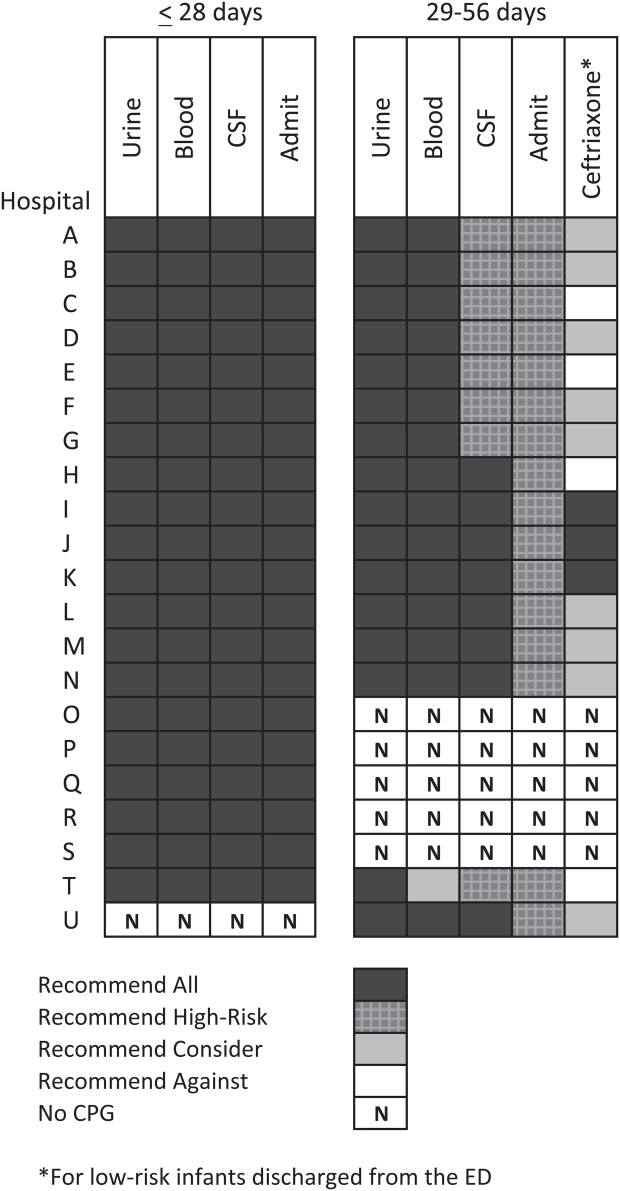
Characteristic | 28 Days | 2956 Days | ||||
---|---|---|---|---|---|---|
No CPG, n=996, N (%) | CPG, n=2,149, N (%) | P Value | No CPG, n=2,460, N (%) | CPG, n=3,772, N (%) | P Value | |
| ||||||
Race | ||||||
Non‐Hispanic white | 325 (32.6) | 996 (46.3) | 867 (35.2) | 1,728 (45.8) | ||
Non‐Hispanic black | 248 (24.9) | 381 (17.7) | 593 (24.1) | 670 (17.8) | ||
Hispanic | 243 (24.4) | 531 (24.7) | 655 (26.6) | 986 (26.1) | ||
Asian | 28 (2.8) | 78 (3.6) | 40 (1.6) | 122 (3.2) | ||
Other Race | 152 (15.3) | 163 (7.6) | <0.001 | 305 (12.4) | 266 (7.1) | <0.001 |
Gender | ||||||
Female | 435 (43.7) | 926 (43.1) | 0.76 | 1,067 (43.4) | 1,714 (45.4) | 0.22 |
Payer | ||||||
Commercial | 243 (24.4) | 738 (34.3) | 554 (22.5) | 1,202 (31.9) | ||
Government | 664 (66.7) | 1,269 (59.1) | 1,798 (73.1) | 2,342 (62.1) | ||
Other payer | 89 (8.9) | 142 (6.6) | <0.001 | 108 (4.4) | 228 (6.0) | <0.001 |
Region | ||||||
Northeast | 39 (3.9) | 245 (11.4) | 77 (3.1) | 572 (15.2) | ||
South | 648 (65.1) | 915 (42.6) | 1,662 (67.6) | 1,462 (38.8) | ||
Midwest | 271 (27.2) | 462 (21.5) | 506 (20.6) | 851 (22.6) | ||
West | 38 (3.8) | 527 (24.5) | <0.001 | 215 (8.7) | 887 (23.5) | <0.001 |
Serious bacterial infection | ||||||
Overall* | 131 (13.2) | 242 (11.3) | 0.14 | 191 (7.8) | 237 (6.3) | 0.03 |
UTI/pyelonephritis | 73 (7.3) | 153 (7.1) | 103 (4.2) | 154 (4.1) | ||
Bacteremia/sepsis | 56 (5.6) | 91 (4.2) | 78 (3.2) | 61 (1.6) | ||
Bacterial meningitis | 15 (1.5) | 15 (0.7) | 4 (0.2) | 14 (0.4) | ||
Age, d, median (IQR) | 18 (11, 24) | 18 (11, 23) | 0.67 | 46 (37, 53) | 45 (37, 53) | 0.11 |
All 20 CPGs for the febrile young infant 28 days of age recommended urine, blood, CSF testing, and hospitalization for all infants (Figure 1). Of the 16 hospitals with CPGs for febrile infants aged 29 to 56 days, all recommended urine and blood testing for all patients, except for 1 CPG, which recommended consideration of blood testing but not to obtain routinely. Hospitals varied in recommendations for CSF testing among infants aged 29 to 56 days: 8 (50%) recommended CSF testing in all patients and 8 (50%) recommended CSF testing only if the patient was high risk per defined criteria (based on history, physical examination, urine, and blood testing). In all 16 CPGs, hospitalization was recommended only for high‐risk infants. For low‐risk infants aged 2956 days being discharged from the ED, 3 hospitals recommended ceftriaxone for all, 9 recommended consideration of ceftriaxone, and 4 recommended against antibiotics (Figure 1).
Study Patients
During the study period, there were 10,415 infants 56 days old with a diagnosis of fever at the 33 participating hospitals. After exclusion of 635 (6.1%) infants with a complex chronic condition and 445 (4.3%) transferred from another institution (including 42 with a complex chronic condition), 9377 infants remained in our study cohort. Approximately one‐third of the cohort was 28 days of age and two‐thirds aged 29 to 56 days. The overall SBI rate was 8.5% but varied by age (11.9% in infants 28 days and 6.9% in infants 29 to 56 days of age) (Table 1).
CPGs and Use of Diagnostic Testing, Hospitalization Rates, Ceftriaxone Use, and Revisits for SBI
For infants 28 days of age, the presence of a CPG was not associated with urine, blood, CSF testing, or hospitalization after multivariable adjustment (Table 2). Among infants aged 29 to 56 days, urine testing did not differ based on the presence of a CPG, whereas blood testing was performed less often at the 1 hospital whose CPG recommended to consider, but not routinely obtain, testing (aOR: 0.4, 95% CI: 0.3‐0.7, P=0.001). Compared to hospitals without a CPG, CSF testing was performed less often at hospitals with CPG recommendations to only obtain CSF if high risk (aOR: 0.5, 95% CI: 0.3‐0.8, P=0.002). However, the odds of hospitalization did not differ at institutions with and without a febrile infant CPG (aOR: 0.7, 95% CI: 0.5‐1.1, P=0.10). For infants aged 29 to 56 days discharged from the ED, ceftriaxone was administered more often at hospitals with CPGs that recommended ceftriaxone for all discharged patients (aOR: 4.6, 95% CI: 2.39.3, P<0.001) and less often at hospitals whose CPGs recommended against antibiotics (aOR: 0.3, 95% CI: 0.1‐0.9, P=0.03) (Table 3). Our findings were similar in the subgroup of infants without bronchiolitis or prematurity (see Supporting Tables 1 and 2 in the online version of this article). After exclusion of hospitals with a CPG implemented during the 2013 calendar year (4 hospitals excluded in the 28 days age group and 2 hospitals excluded in the 29 to 56 days age group), infants aged 29 to 56 days cared for at a hospital with a CPG experienced a lower odds of hospitalization (aOR: 0.7, 95% CI: 0.4‐0.98, P=0.04). Otherwise, our findings in both age groups did not materially differ from the main analyses.
Testing/Hospitalization | No. of Hospitals | No. of Patients | % Received* | aOR (95% CI) | P Value |
---|---|---|---|---|---|
| |||||
Laboratory testing | |||||
Urine testing | |||||
No CPG | 13 | 996 | 75.6 | Ref | |
CPG: recommend for all | 20 | 2,149 | 80.7 | 1.2 (0.9‐1.7) | 0.22 |
Blood testing | |||||
No CPG | 13 | 996 | 76.9 | Ref | |
CPG: recommend for all | 20 | 2,149 | 81.8 | 1.2 (0.9‐1.7) | 0.25 |
CSF testing‖ | |||||
No CPG | 13 | 996 | 71.0 | Ref | |
CPG: recommend for all | 20 | 2,149 | 77.5 | 1.3 (1.01.7) | 0.08 |
Disposition | |||||
Hospitalization | |||||
No CPG | 13 | 996 | 75.4 | Ref | |
CPG: recommend for all | 20 | 2,149 | 81.6 | 1.2 (0.9‐1.8) | 0.26 |
Testing/Hospitalization | No. of Hospitals | No. of Patients | % Received* | aOR (95% CI) | P Value |
---|---|---|---|---|---|
| |||||
Laboratory resting | |||||
Urine testing | |||||
No CPG | 17 | 2,460 | 81.1 | Ref | |
CPG: recommend for all | 16 | 3,772 | 82.1 | 0.9 (0.7‐1.4) | 0.76 |
Blood testing | |||||
No CPG | 17 | 2,460 | 79.4 | Ref | |
CPG: recommend for all | 15 | 3,628 | 82.6 | 1.1 (0.7‐1.6) | 0.70 |
CPG: recommend consider | 1 | 144 | 62.5 | 0.4 (0.3‐0.7) | 0.001 |
CSF testing‖ | |||||
No CPG | 17 | 2,460 | 46.3 | Ref | |
CPG: recommend for all | 8 | 1,517 | 70.3 | 1.3 (0.9‐1.9) | 0.11 |
CPG: recommend if high‐risk | 8 | 2,255 | 39.9 | 0.5 (0.3‐0.8) | 0.002 |
Disposition | |||||
Hospitalization | |||||
No CPG | 17 | 2,460 | 47.0 | Ref | |
CPG: recommend if high‐risk | 16 | 3,772 | 42.0 | 0.7 (0.5‐1.1) | 0.10 |
Ceftriaxone if discharged | |||||
No CPG | 17 | 1,304 | 11.7 | Ref | |
CPG: recommend against | 4 | 313 | 10.9 | 0.3 (0.1‐0.9) | 0.03 |
CPG: recommend consider | 9 | 1,567 | 14.4 | 1.5 (0.9‐2.4) | 0.09 |
CPG: recommend for all | 3 | 306 | 64.1 | 4.6 (2.39.3) | < 0.001 |
Three‐day revisits for SBI were similarly low at hospitals with and without CPGs among infants 28 days (1.5% vs 0.8%, P=0.44) and 29 to 56 days of age (1.4% vs 1.1%, P=0.44) and did not differ after exclusion of hospitals with a CPG implemented in 2013.
CPGs and Costs
Among infants 28 days of age, costs per visit did not differ for admitted and discharged patients based on CPG presence. The presence of an ED febrile infant CPG was associated with higher costs for both admitted and discharged infants 29 to 56 days of age (Table 4). The cost analysis did not significantly differ after exclusion of hospitals with CPGs implemented in 2013.
28 Days, Cost, Median (IQR) | 29 to 56 Days, Cost, Median (IQR) | |||||
---|---|---|---|---|---|---|
No CPG | CPG | P Value | No CPG | CPG | P Value | |
| ||||||
Admitted | $4,979 ($3,408$6,607) [n=751] | $4,715 ($3,472$6,526) [n=1,753] | 0.79 | $3,756 ($2,725$5,041) [n=1,156] | $3,923 ($3,077$5,243) [n=1,586] | <0.001 |
Discharged | $298 ($166$510) [n=245] | $231 ($160$464) [n=396] | 0.10 | $681($398$982) [n=1,304)] | $764 ($412$1,100) [n=2,186] | <0.001 |
DISCUSSION
We described the content and association of CPGs with management of the febrile infant 56 days of age across a large sample of children's hospitals. Nearly two‐thirds of included pediatric EDs have a CPG for the management of young febrile infants. Management of febrile infants 28 days was uniform, with a majority hospitalized after urine, blood, and CSF testing regardless of the presence of a CPG. In contrast, CPGs for infants 29 to 56 days of age varied in their recommendations for CSF testing as well as ceftriaxone use for infants discharged from the ED. Consequently, we observed considerable hospital variability in CSF testing and ceftriaxone use for discharged infants, which correlates with variation in the presence and content of CPGs. Institutional CPGs may be a source of the across‐hospital variation in care of febrile young infants observed in prior study.[9]
Febrile infants 28 days of age are at particularly high risk for SBI, with a prevalence of nearly 20% or higher.[2, 3, 29] The high prevalence of SBI, combined with the inherent difficulty in distinguishing neonates with and without SBI,[2, 30] has resulted in uniform CPG recommendations to perform the full‐sepsis workup in this young age group. Similar to prior studies,[8, 9] we observed that most febrile infants 28 days undergo the full sepsis evaluation, including CSF testing, and are hospitalized regardless of the presence of a CPG.
However, given the conflicting recommendations for febrile infants 29 to 56 days of age,[4, 5, 6] the optimal management strategy is less certain.[7] The Rochester, Philadelphia, and Boston criteria, 3 published models to identify infants at low risk for SBI, primarily differ in their recommendations for CSF testing and ceftriaxone use in this age group.[4, 5, 6] Half of the CPGs recommended CSF testing for all febrile infants, and half recommended CSF testing only if the infant was high risk. Institutional guidelines that recommended selective CSF testing for febrile infants aged 29 to 56 days were associated with lower rates of CSF testing. Furthermore, ceftriaxone use varied based on CPG recommendations for low‐risk infants discharged from the ED. Therefore, the influence of febrile infant CPGs mainly relates to the limiting of CSF testing and targeted ceftriaxone use in low‐risk infants. As the rate of return visits for SBI is low across hospitals, future study should assess outcomes at hospitals with CPGs recommending selective CSF testing. Of note, infants 29 to 56 days of age were less likely to be hospitalized when cared for at a hospital with an established CPG prior to 2013 without increase in 3‐day revisits for SBI. This finding may indicate that longer duration of CPG implementation is associated with lower rates of hospitalization for low‐risk infants; this finding merits further study.
The presence of a CPG was not associated with lower costs for febrile infants in either age group. Although individual healthcare systems have achieved lower costs with CPG implementation,[12] the mere presence of a CPG is not associated with lower costs when assessed across institutions. Higher costs for admitted and discharged infants 29 to 56 days of age in the presence of a CPG likely reflects the higher rate of CSF testing at hospitals whose CPGs recommend testing for all febrile infants, as well as inpatient management strategies for hospitalized infants not captured in our study. Future investigation should include an assessment of the cost‐effectiveness of the various testing and treatment strategies employed for the febrile young infant.
Our study has several limitations. First, the validity of ICD‐9 diagnosis codes for identifying young infants with fever is not well established, and thus our study is subject to misclassification bias. To minimize missed patients, we included infants with either an ICD‐9 admission or discharge diagnosis of fever; however, utilization of diagnosis codes for patient identification may have resulted in undercapture of infants with a measured temperature of 38.0C. It is also possible that some patients who did not undergo testing were misclassified as having a fever or had temperatures below standard thresholds to prompt diagnostic testing. This is a potential reason that testing was not performed in 100% of infants, even at hospitals with CPGs that recommended testing for all patients. Additionally, some febrile infants diagnosed with SBI may not have an associated ICD‐9 diagnosis code for fever. Although the overall SBI rate observed in our study was similar to prior studies,[4, 31] the rate in neonates 28 days of age was lower than reported in recent investigations,[2, 3] which may indicate inclusion of a higher proportion of low‐risk febrile infants. With the exception of bronchiolitis, we also did not assess diagnostic testing in the presence of other identified sources of infection such as herpes simplex virus.
Second, we were unable to assess the presence or absence of a CPG at the 4 excluded EDs that did not respond to the survey or the institutions excluded for data‐quality issues. However, included and excluded hospitals did not differ in region or annual ED volume (data not shown).
Third, although we classified hospitals based upon the presence and content of CPGs, we were unable to fully evaluate adherence to the CPG at each site.
Last, though PHIS hospitals represent 85% of freestanding children's hospitals, many febrile infants are hospitalized at non‐PHIS institutions; our results may not be generalizable to care provided at nonchildren's hospitals.
CONCLUSIONS
Management of febrile neonates 28 days of age does not vary based on CPG presence. However, CPGs for the febrile infant aged 29 to 56 days vary in recommendations for CSF testing as well as ceftriaxone use for low‐risk patients, which significantly contributes to practice variation and healthcare costs across institutions.
Acknowledgements
The Febrile Young Infant Research Collaborative includes the following additional investigators who are acknowledged for their work on this study: Kao‐Ping Chua, MD, Harvard PhD Program in Health Policy, Harvard University, Cambridge, Massachusetts, and Division of Emergency Medicine, Department of Pediatrics, Boston Children's Hospital, Boston, Massachusetts; Elana A. Feldman, BA, University of Washington School of Medicine, Seattle, Washington; and Katie L. Hayes, BS, Division of Emergency Medicine, Department of Pediatrics, The Children's Hospital of Philadelphia, Philadelphia, Pennsylvania.
Disclosures
This project was funded in part by The Gerber Foundation Novice Researcher Award (Ref #18273835). Dr. Fran Balamuth received career development support from the National Institutes of Health (NHLBI K12‐HL109009). Funders were not involved in design or conduct of the study; collection, management, analysis, or interpretation of the data; preparation, review, or approval of the manuscript. The authors have no financial relationships relevant to this article to disclose. No payment was received for the production of this article. The authors have no conflicts of interest relevant to this article to disclose.
Febrile young infants are at high risk for serious bacterial infection (SBI) with reported rates of 8.5% to 12%, even higher in neonates 28 days of age.[1, 2, 3] As a result, febrile infants often undergo extensive diagnostic evaluation consisting of a combination of urine, blood, and cerebrospinal fluid (CSF) testing.[4, 5, 6] Several clinical prediction algorithms use this diagnostic testing to identify febrile infants at low risk for SBI, but they differ with respect to age range, recommended testing, antibiotic administration, and threshold for hospitalization.[4, 5, 6] Additionally, the optimal management strategy for this population has not been defined.[7] Consequently, laboratory testing, antibiotic use, and hospitalization for febrile young infants vary widely among hospitals.[8, 9, 10]
Clinical practice guidelines (CPGs) are designed to implement evidence‐based care and reduce practice variability, with the goal of improving quality of care and optimizing costs.[11] Implementation of a CPG for management of febrile young infants in the Intermountain Healthcare System was associated with greater adherence to evidence‐based care and lower costs.[12] However, when strong evidence is lacking, different interpretations of febrile infant risk classification incorporated into local CPGs may be a major driver of the across‐hospital practice variation observed in prior studies.[8, 9] Understanding sources of variability as well as determining the association of CPGs with clinicians' practice patterns can help identify quality improvement opportunities, either through national benchmarking or local efforts.
Our primary objectives were to compare (1) recommendations of pediatric emergency departmentbased institutional CPGs for febrile young infants and (2) rates of urine, blood, CSF testing, hospitalization, and ceftriaxone use at emergency department (ED) discharge based upon CPG presence and the specific CPG recommendations. Our secondary objectives were to describe the association of CPGs with healthcare costs and return visits for SBI.
METHODS
Study Design
We used the Pediatric Health Information System (PHIS) to identify febrile infants 56 days of age who presented to the ED between January 1, 2013 and December 31, 2013. We also surveyed ED providers at participating PHIS hospitals. Informed consent was obtained from survey respondents. The institutional review board at Boston Children's Hospital approved the study protocol.
Clinical Practice Guideline Survey
We sent an electronic survey to medical directors or division directors at 37 pediatric EDs to determine whether their ED utilized a CPG for the management of the febrile young infant in 2013. If no response was received after the second attempt, we queried ED fellowship directors or other ED attending physicians at nonresponding hospitals. Survey items included the presence of a febrile young infant CPG, and if present, the year of implementation, ages targeted, and CPG content. As applicable, respondents were asked to share their CPG and/or provide the specific CPG recommendations.
We collected and managed survey data using the Research Electronic Data Capture (REDCap) electronic data capture tools hosted at Boston Children's Hospital. REDCap is a secure, Web‐based application designed to support data capture for research studies.[13]
Data Source
The PHIS database contains administrative data from 44 US children's hospitals. These hospitals, affiliated with the Children's Hospital Association, represent 85% of freestanding US children's hospitals.[14] Encrypted patient identifiers permit tracking of patients across encounters.[15] Data quality and integrity are assured jointly by the Children's Hospital Association and participating hospitals.[16] For this study, 7 hospitals were excluded due to incomplete ED data or known data‐quality issues.[17]
Patients
We identified study infants using the following International Classification of Diseases, 9th Revision (ICD‐9) admission or discharge diagnosis codes for fever as defined previously[8, 9]: 780.6, 778.4, 780.60, or 780.61. We excluded infants with a complex chronic condition[18] and those transferred from another institution, as these infants may warrant a nonstandard evaluation and/or may have incomplete data. For infants with >1 ED visit for fever during the study period, repeat visits within 3 days of an index visit were considered a revisit for the same episode of illness; visits >3 days following an index visit were considered as a new index visit.
Study Definitions
From the PHIS database, we abstracted demographic characteristics (gender, race/ethnicity), insurance status, and region where the hospital was located (using US Census categories[19]). Billing codes were used to assess whether urine, blood, and CSF testing (as defined previously[9]) were performed during the ED evaluation. To account for ED visits that spanned the midnight hour, for hospitalized patients we considered any testing or treatment occurring on the initial or second hospital day to be performed in the ED; billing code data in PHIS are based upon calendar day and do not distinguish testing performed in the ED versus inpatient setting.[8, 9] Patients billed for observation care were classified as being hospitalized.[20, 21]
We identified the presence of an SBI using ICD‐9 diagnosis codes for the following infections as described previously[9]: urinary tract infection or pyelonephritis,[22] bacteremia or sepsis, bacterial meningitis,[16] pneumonia,[23] or bacterial enteritis. To assess return visits for SBI that required inpatient management, we defined an ED revisit for an SBI as a return visit within 3 days of ED discharge[24, 25] that resulted in hospitalization with an associated ICD‐9 discharge diagnosis code for an SBI.
Hospitals charges in PHIS database were adjusted for hospital location by using the Centers for Medicare and Medicaid Services price/wage index. Costs were estimated by applying hospital‐level cost‐to‐charge ratios to charge data.[26]
Measured Exposures
The primary exposure was the presence of an ED‐based CPG for management of the febrile young infant aged 28 days and 29 to 56 days; 56 days was used as the upper age limit as all of the CPGs included infants up to this age or beyond. Six institutions utilized CPGs with different thresholds to define the age categories (eg, dichotomized at 27 or 30 days); these CPGs were classified into the aforementioned age groups to permit comparisons across standardized age groups. We classified institutions based on the presence of a CPG. To assess differences in the application of low‐risk criteria, the CPGs were further classified a priori based upon specific recommendations around laboratory testing and hospitalization, as well as ceftriaxone use for infants aged 29 to 56 days discharged from the ED. CPGs were categorized based upon whether testing, hospitalization, and ceftriaxone use were: (1) recommended for all patients, (2) recommended only if patients were classified as high risk (absence of low‐risk criteria), (3) recommended against, or (4) recommended to consider at clinician discretion.
Outcome Measures
Measured outcomes were performance of urine, blood, CSF testing, and hospitalization rate, as well as rate of ceftriaxone use for discharged infants aged 29 to 56 days, 3‐day revisits for SBI, and costs per visit, which included hospitalization costs for admitted patients.
Data Analysis
We described continuous variables using median and interquartile range or range values and categorical variables using frequencies. We compared medians using Wilcoxon rank sum and categorical variables using a [2] test. We compared rates of testing, hospitalization, ceftriaxone use, and 3‐day revisits for SBI based on the presence of a CPG, and when present, the specific CPG recommendations. Costs per visit were compared between institutions with and without CPGs and assessed separately for admitted and discharged patients. To adjust for potential confounders and clustering of patients within hospitals, we used generalized estimating equations with logistic regression to generate adjusted odd ratios (aORs) and 95% confidence intervals (CIs). Models were adjusted for geographic region, payer, race, and gender. Statistical analyses were performed by using SAS version 9.3 (SAS Institute, Cary, NC). We determined statistical significance as a 2‐tailed P value <0.05.
Febrile infants with bronchiolitis or a history of prematurity may be managed differently from full‐term febrile young infants without bronchiolitis.[6, 27] Therefore, we performed a subgroup analysis after exclusion of infants with an ICD‐9 discharge diagnosis code for bronchiolitis (466.11 and 466.19)[28] or prematurity (765).
Because our study included ED encounters in 2013, we repeated our analyses after exclusion of hospitals with CPGs implemented during the 2013 calendar year.
RESULTS
CPG by Institution
Thirty‐three (89.2%) of the 37 EDs surveyed completed the questionnaire. Overall, 21 (63.6%) of the 33 EDs had a CPG; 15 (45.5%) had a CPG for all infants 56 days of age, 5 (15.2%) had a CPG for infants 28 days only, and 1 (3.0%) had a CPG for infants 29 to 56 days but not 28 days of age (Figure 1). Seventeen EDs had an established CPG prior to 2013, and 4 hospitals implemented a CPG during the 2013 calendar year, 2 with CPGs for neonates 28 days and 2 with CPGs for both 28 days and 29 to 56 days of age. Hospitals with CPGs were more likely to be located in the Northeast and West regions of the United States and provide care to a higher proportion of non‐Hispanic white patients, as well as those with commercial insurance (Table 1).
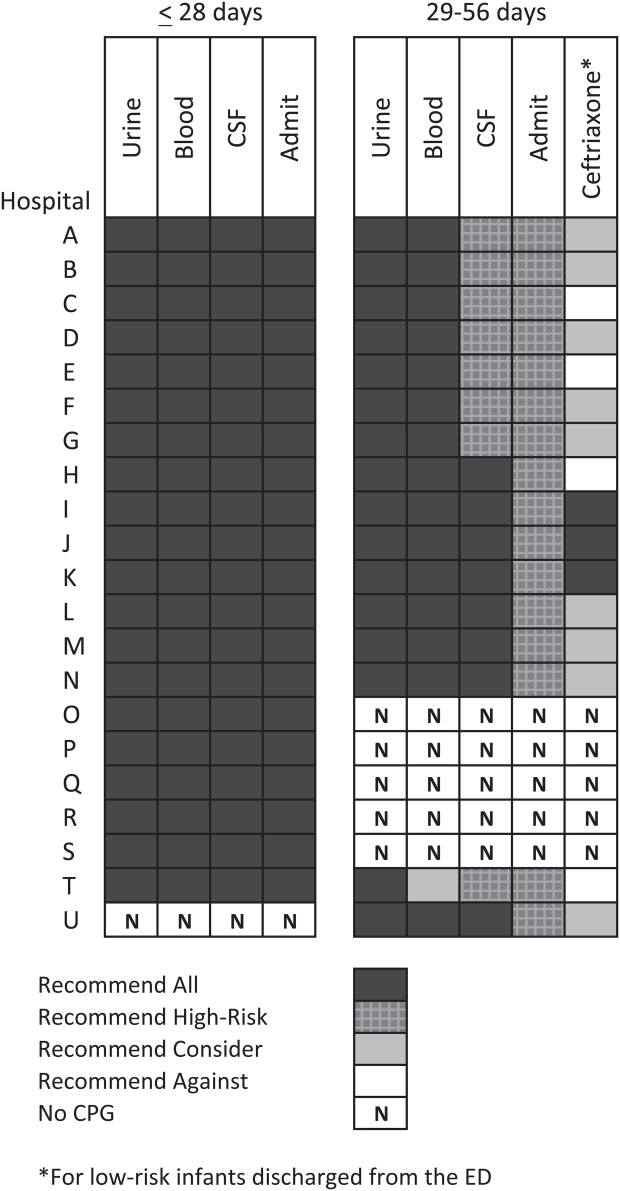
Characteristic | 28 Days | 2956 Days | ||||
---|---|---|---|---|---|---|
No CPG, n=996, N (%) | CPG, n=2,149, N (%) | P Value | No CPG, n=2,460, N (%) | CPG, n=3,772, N (%) | P Value | |
| ||||||
Race | ||||||
Non‐Hispanic white | 325 (32.6) | 996 (46.3) | 867 (35.2) | 1,728 (45.8) | ||
Non‐Hispanic black | 248 (24.9) | 381 (17.7) | 593 (24.1) | 670 (17.8) | ||
Hispanic | 243 (24.4) | 531 (24.7) | 655 (26.6) | 986 (26.1) | ||
Asian | 28 (2.8) | 78 (3.6) | 40 (1.6) | 122 (3.2) | ||
Other Race | 152 (15.3) | 163 (7.6) | <0.001 | 305 (12.4) | 266 (7.1) | <0.001 |
Gender | ||||||
Female | 435 (43.7) | 926 (43.1) | 0.76 | 1,067 (43.4) | 1,714 (45.4) | 0.22 |
Payer | ||||||
Commercial | 243 (24.4) | 738 (34.3) | 554 (22.5) | 1,202 (31.9) | ||
Government | 664 (66.7) | 1,269 (59.1) | 1,798 (73.1) | 2,342 (62.1) | ||
Other payer | 89 (8.9) | 142 (6.6) | <0.001 | 108 (4.4) | 228 (6.0) | <0.001 |
Region | ||||||
Northeast | 39 (3.9) | 245 (11.4) | 77 (3.1) | 572 (15.2) | ||
South | 648 (65.1) | 915 (42.6) | 1,662 (67.6) | 1,462 (38.8) | ||
Midwest | 271 (27.2) | 462 (21.5) | 506 (20.6) | 851 (22.6) | ||
West | 38 (3.8) | 527 (24.5) | <0.001 | 215 (8.7) | 887 (23.5) | <0.001 |
Serious bacterial infection | ||||||
Overall* | 131 (13.2) | 242 (11.3) | 0.14 | 191 (7.8) | 237 (6.3) | 0.03 |
UTI/pyelonephritis | 73 (7.3) | 153 (7.1) | 103 (4.2) | 154 (4.1) | ||
Bacteremia/sepsis | 56 (5.6) | 91 (4.2) | 78 (3.2) | 61 (1.6) | ||
Bacterial meningitis | 15 (1.5) | 15 (0.7) | 4 (0.2) | 14 (0.4) | ||
Age, d, median (IQR) | 18 (11, 24) | 18 (11, 23) | 0.67 | 46 (37, 53) | 45 (37, 53) | 0.11 |
All 20 CPGs for the febrile young infant 28 days of age recommended urine, blood, CSF testing, and hospitalization for all infants (Figure 1). Of the 16 hospitals with CPGs for febrile infants aged 29 to 56 days, all recommended urine and blood testing for all patients, except for 1 CPG, which recommended consideration of blood testing but not to obtain routinely. Hospitals varied in recommendations for CSF testing among infants aged 29 to 56 days: 8 (50%) recommended CSF testing in all patients and 8 (50%) recommended CSF testing only if the patient was high risk per defined criteria (based on history, physical examination, urine, and blood testing). In all 16 CPGs, hospitalization was recommended only for high‐risk infants. For low‐risk infants aged 2956 days being discharged from the ED, 3 hospitals recommended ceftriaxone for all, 9 recommended consideration of ceftriaxone, and 4 recommended against antibiotics (Figure 1).
Study Patients
During the study period, there were 10,415 infants 56 days old with a diagnosis of fever at the 33 participating hospitals. After exclusion of 635 (6.1%) infants with a complex chronic condition and 445 (4.3%) transferred from another institution (including 42 with a complex chronic condition), 9377 infants remained in our study cohort. Approximately one‐third of the cohort was 28 days of age and two‐thirds aged 29 to 56 days. The overall SBI rate was 8.5% but varied by age (11.9% in infants 28 days and 6.9% in infants 29 to 56 days of age) (Table 1).
CPGs and Use of Diagnostic Testing, Hospitalization Rates, Ceftriaxone Use, and Revisits for SBI
For infants 28 days of age, the presence of a CPG was not associated with urine, blood, CSF testing, or hospitalization after multivariable adjustment (Table 2). Among infants aged 29 to 56 days, urine testing did not differ based on the presence of a CPG, whereas blood testing was performed less often at the 1 hospital whose CPG recommended to consider, but not routinely obtain, testing (aOR: 0.4, 95% CI: 0.3‐0.7, P=0.001). Compared to hospitals without a CPG, CSF testing was performed less often at hospitals with CPG recommendations to only obtain CSF if high risk (aOR: 0.5, 95% CI: 0.3‐0.8, P=0.002). However, the odds of hospitalization did not differ at institutions with and without a febrile infant CPG (aOR: 0.7, 95% CI: 0.5‐1.1, P=0.10). For infants aged 29 to 56 days discharged from the ED, ceftriaxone was administered more often at hospitals with CPGs that recommended ceftriaxone for all discharged patients (aOR: 4.6, 95% CI: 2.39.3, P<0.001) and less often at hospitals whose CPGs recommended against antibiotics (aOR: 0.3, 95% CI: 0.1‐0.9, P=0.03) (Table 3). Our findings were similar in the subgroup of infants without bronchiolitis or prematurity (see Supporting Tables 1 and 2 in the online version of this article). After exclusion of hospitals with a CPG implemented during the 2013 calendar year (4 hospitals excluded in the 28 days age group and 2 hospitals excluded in the 29 to 56 days age group), infants aged 29 to 56 days cared for at a hospital with a CPG experienced a lower odds of hospitalization (aOR: 0.7, 95% CI: 0.4‐0.98, P=0.04). Otherwise, our findings in both age groups did not materially differ from the main analyses.
Testing/Hospitalization | No. of Hospitals | No. of Patients | % Received* | aOR (95% CI) | P Value |
---|---|---|---|---|---|
| |||||
Laboratory testing | |||||
Urine testing | |||||
No CPG | 13 | 996 | 75.6 | Ref | |
CPG: recommend for all | 20 | 2,149 | 80.7 | 1.2 (0.9‐1.7) | 0.22 |
Blood testing | |||||
No CPG | 13 | 996 | 76.9 | Ref | |
CPG: recommend for all | 20 | 2,149 | 81.8 | 1.2 (0.9‐1.7) | 0.25 |
CSF testing‖ | |||||
No CPG | 13 | 996 | 71.0 | Ref | |
CPG: recommend for all | 20 | 2,149 | 77.5 | 1.3 (1.01.7) | 0.08 |
Disposition | |||||
Hospitalization | |||||
No CPG | 13 | 996 | 75.4 | Ref | |
CPG: recommend for all | 20 | 2,149 | 81.6 | 1.2 (0.9‐1.8) | 0.26 |
Testing/Hospitalization | No. of Hospitals | No. of Patients | % Received* | aOR (95% CI) | P Value |
---|---|---|---|---|---|
| |||||
Laboratory resting | |||||
Urine testing | |||||
No CPG | 17 | 2,460 | 81.1 | Ref | |
CPG: recommend for all | 16 | 3,772 | 82.1 | 0.9 (0.7‐1.4) | 0.76 |
Blood testing | |||||
No CPG | 17 | 2,460 | 79.4 | Ref | |
CPG: recommend for all | 15 | 3,628 | 82.6 | 1.1 (0.7‐1.6) | 0.70 |
CPG: recommend consider | 1 | 144 | 62.5 | 0.4 (0.3‐0.7) | 0.001 |
CSF testing‖ | |||||
No CPG | 17 | 2,460 | 46.3 | Ref | |
CPG: recommend for all | 8 | 1,517 | 70.3 | 1.3 (0.9‐1.9) | 0.11 |
CPG: recommend if high‐risk | 8 | 2,255 | 39.9 | 0.5 (0.3‐0.8) | 0.002 |
Disposition | |||||
Hospitalization | |||||
No CPG | 17 | 2,460 | 47.0 | Ref | |
CPG: recommend if high‐risk | 16 | 3,772 | 42.0 | 0.7 (0.5‐1.1) | 0.10 |
Ceftriaxone if discharged | |||||
No CPG | 17 | 1,304 | 11.7 | Ref | |
CPG: recommend against | 4 | 313 | 10.9 | 0.3 (0.1‐0.9) | 0.03 |
CPG: recommend consider | 9 | 1,567 | 14.4 | 1.5 (0.9‐2.4) | 0.09 |
CPG: recommend for all | 3 | 306 | 64.1 | 4.6 (2.39.3) | < 0.001 |
Three‐day revisits for SBI were similarly low at hospitals with and without CPGs among infants 28 days (1.5% vs 0.8%, P=0.44) and 29 to 56 days of age (1.4% vs 1.1%, P=0.44) and did not differ after exclusion of hospitals with a CPG implemented in 2013.
CPGs and Costs
Among infants 28 days of age, costs per visit did not differ for admitted and discharged patients based on CPG presence. The presence of an ED febrile infant CPG was associated with higher costs for both admitted and discharged infants 29 to 56 days of age (Table 4). The cost analysis did not significantly differ after exclusion of hospitals with CPGs implemented in 2013.
28 Days, Cost, Median (IQR) | 29 to 56 Days, Cost, Median (IQR) | |||||
---|---|---|---|---|---|---|
No CPG | CPG | P Value | No CPG | CPG | P Value | |
| ||||||
Admitted | $4,979 ($3,408$6,607) [n=751] | $4,715 ($3,472$6,526) [n=1,753] | 0.79 | $3,756 ($2,725$5,041) [n=1,156] | $3,923 ($3,077$5,243) [n=1,586] | <0.001 |
Discharged | $298 ($166$510) [n=245] | $231 ($160$464) [n=396] | 0.10 | $681($398$982) [n=1,304)] | $764 ($412$1,100) [n=2,186] | <0.001 |
DISCUSSION
We described the content and association of CPGs with management of the febrile infant 56 days of age across a large sample of children's hospitals. Nearly two‐thirds of included pediatric EDs have a CPG for the management of young febrile infants. Management of febrile infants 28 days was uniform, with a majority hospitalized after urine, blood, and CSF testing regardless of the presence of a CPG. In contrast, CPGs for infants 29 to 56 days of age varied in their recommendations for CSF testing as well as ceftriaxone use for infants discharged from the ED. Consequently, we observed considerable hospital variability in CSF testing and ceftriaxone use for discharged infants, which correlates with variation in the presence and content of CPGs. Institutional CPGs may be a source of the across‐hospital variation in care of febrile young infants observed in prior study.[9]
Febrile infants 28 days of age are at particularly high risk for SBI, with a prevalence of nearly 20% or higher.[2, 3, 29] The high prevalence of SBI, combined with the inherent difficulty in distinguishing neonates with and without SBI,[2, 30] has resulted in uniform CPG recommendations to perform the full‐sepsis workup in this young age group. Similar to prior studies,[8, 9] we observed that most febrile infants 28 days undergo the full sepsis evaluation, including CSF testing, and are hospitalized regardless of the presence of a CPG.
However, given the conflicting recommendations for febrile infants 29 to 56 days of age,[4, 5, 6] the optimal management strategy is less certain.[7] The Rochester, Philadelphia, and Boston criteria, 3 published models to identify infants at low risk for SBI, primarily differ in their recommendations for CSF testing and ceftriaxone use in this age group.[4, 5, 6] Half of the CPGs recommended CSF testing for all febrile infants, and half recommended CSF testing only if the infant was high risk. Institutional guidelines that recommended selective CSF testing for febrile infants aged 29 to 56 days were associated with lower rates of CSF testing. Furthermore, ceftriaxone use varied based on CPG recommendations for low‐risk infants discharged from the ED. Therefore, the influence of febrile infant CPGs mainly relates to the limiting of CSF testing and targeted ceftriaxone use in low‐risk infants. As the rate of return visits for SBI is low across hospitals, future study should assess outcomes at hospitals with CPGs recommending selective CSF testing. Of note, infants 29 to 56 days of age were less likely to be hospitalized when cared for at a hospital with an established CPG prior to 2013 without increase in 3‐day revisits for SBI. This finding may indicate that longer duration of CPG implementation is associated with lower rates of hospitalization for low‐risk infants; this finding merits further study.
The presence of a CPG was not associated with lower costs for febrile infants in either age group. Although individual healthcare systems have achieved lower costs with CPG implementation,[12] the mere presence of a CPG is not associated with lower costs when assessed across institutions. Higher costs for admitted and discharged infants 29 to 56 days of age in the presence of a CPG likely reflects the higher rate of CSF testing at hospitals whose CPGs recommend testing for all febrile infants, as well as inpatient management strategies for hospitalized infants not captured in our study. Future investigation should include an assessment of the cost‐effectiveness of the various testing and treatment strategies employed for the febrile young infant.
Our study has several limitations. First, the validity of ICD‐9 diagnosis codes for identifying young infants with fever is not well established, and thus our study is subject to misclassification bias. To minimize missed patients, we included infants with either an ICD‐9 admission or discharge diagnosis of fever; however, utilization of diagnosis codes for patient identification may have resulted in undercapture of infants with a measured temperature of 38.0C. It is also possible that some patients who did not undergo testing were misclassified as having a fever or had temperatures below standard thresholds to prompt diagnostic testing. This is a potential reason that testing was not performed in 100% of infants, even at hospitals with CPGs that recommended testing for all patients. Additionally, some febrile infants diagnosed with SBI may not have an associated ICD‐9 diagnosis code for fever. Although the overall SBI rate observed in our study was similar to prior studies,[4, 31] the rate in neonates 28 days of age was lower than reported in recent investigations,[2, 3] which may indicate inclusion of a higher proportion of low‐risk febrile infants. With the exception of bronchiolitis, we also did not assess diagnostic testing in the presence of other identified sources of infection such as herpes simplex virus.
Second, we were unable to assess the presence or absence of a CPG at the 4 excluded EDs that did not respond to the survey or the institutions excluded for data‐quality issues. However, included and excluded hospitals did not differ in region or annual ED volume (data not shown).
Third, although we classified hospitals based upon the presence and content of CPGs, we were unable to fully evaluate adherence to the CPG at each site.
Last, though PHIS hospitals represent 85% of freestanding children's hospitals, many febrile infants are hospitalized at non‐PHIS institutions; our results may not be generalizable to care provided at nonchildren's hospitals.
CONCLUSIONS
Management of febrile neonates 28 days of age does not vary based on CPG presence. However, CPGs for the febrile infant aged 29 to 56 days vary in recommendations for CSF testing as well as ceftriaxone use for low‐risk patients, which significantly contributes to practice variation and healthcare costs across institutions.
Acknowledgements
The Febrile Young Infant Research Collaborative includes the following additional investigators who are acknowledged for their work on this study: Kao‐Ping Chua, MD, Harvard PhD Program in Health Policy, Harvard University, Cambridge, Massachusetts, and Division of Emergency Medicine, Department of Pediatrics, Boston Children's Hospital, Boston, Massachusetts; Elana A. Feldman, BA, University of Washington School of Medicine, Seattle, Washington; and Katie L. Hayes, BS, Division of Emergency Medicine, Department of Pediatrics, The Children's Hospital of Philadelphia, Philadelphia, Pennsylvania.
Disclosures
This project was funded in part by The Gerber Foundation Novice Researcher Award (Ref #18273835). Dr. Fran Balamuth received career development support from the National Institutes of Health (NHLBI K12‐HL109009). Funders were not involved in design or conduct of the study; collection, management, analysis, or interpretation of the data; preparation, review, or approval of the manuscript. The authors have no financial relationships relevant to this article to disclose. No payment was received for the production of this article. The authors have no conflicts of interest relevant to this article to disclose.
- Performance of low‐risk criteria in the evaluation of young infants with fever: review of the literature. Pediatrics. 2010;125:228–233. , , .
- A week‐by‐week analysis of the low‐risk criteria for serious bacterial infection in febrile neonates. Arch Dis Child. 2009;94:287–292. , , , , , .
- Is 15 days an appropriate cut‐off age for considering serious bacterial infection in the management of febrile infants? Pediatr Infect Dis J. 2012;31:455–458. , , , et al.
- Outpatient management without antibiotics of fever in selected infants. N Engl J Med. 1993;329:1437–1441. , , .
- Identifying febrile infants at risk for a serious bacterial infection. J Pediatr. 1993;123:489–490. , , .
- Febrile infants at low risk for serious bacterial infection—an appraisal of the Rochester criteria and implications for management. Febrile Infant Collaborative Study Group. Pediatrics. 1994;94:390–396. , , , et al.
- American College of Emergency Physicians Clinical Policies Committee; American College of Emergency Physicians Clinical Policies Subcommittee on Pediatric Fever. Clinical policy for children younger than three years presenting to the emergency department with fever. Ann Emerg Med. 2003;42:530–545.
- Management of febrile neonates in US pediatric emergency departments. Pediatrics. 2014;133:187–195. , , , et al.
- Variation in care of the febrile young infant <90 days in US pediatric emergency departments. Pediatrics. 2014;134:667–677. , , , et al.
- Fever survey highlights significant variations in how infants aged ≤60 days are evaluated and underline the need for guidelines. Acta Paediatr. 2014;103:379–385. , , , , .
- Evidence‐based guidelines and critical pathways for quality improvement. Pediatrics. 1999;103:225–232. .
- Costs and infant outcomes after implementation of a care process model for febrile infants. Pediatrics. 2012;130:e16–e24. , , , et al.
- Research electronic data capture (REDCap)—a metadata‐driven methodology and workflow process for providing translational research informatics support. J Biomed Inform. 2009;42:377–381. , , , , , .
- Variation in occult injury screening for children with suspected abuse in selected US children's hospitals. Pediatrics. 2012;130:853–860. , , , , , .
- Achieving data quality. How data from a pediatric health information system earns the trust of its users. J AHIMA. 2004;75:22–26. .
- Corticosteroids and mortality in children with bacterial meningitis. JAMA. 2008;299:2048–2055. , , , .
- Variation in resource utilization across a national sample of pediatric emergency departments. J Pediatr. 2013;163:230–236. , , , et al.
- Deaths attributed to pediatric complex chronic conditions: national trends and implications for supportive care services. Pediatrics. 2001;107:E99. , , , , , .
- US Census Bureau. Geographic terms and concepts—census divisions and census regions. Available at: https://www.census.gov/geo/reference/gtc/gtc_census_divreg.html. Accessed September 10, 2014.
- Pediatric observation status: are we overlooking a growing population in children's hospitals? J Hosp Med. 2012;7:530–536. , , , et al.
- Differences in designations of observation care in US freestanding children's hospitals: are they virtual or real? J Hosp Med. 2012;7:287–293. , , , et al.
- Accuracy of administrative billing codes to detect urinary tract infection hospitalizations. Pediatrics. 2011;128:323–330. , , , et al.
- Identifying pediatric community‐acquired pneumonia hospitalizations: accuracy of administrative billing codes. JAMA Pediatr. 2013;167:851–858. , , , et al.
- Initial emergency department diagnosis and return visits: risk versus perception. Ann Emerg Med. 1998;32:569–573. , , , .
- A national depiction of children with return visits to the emergency department within 72 hours, 2001–2007. Pediatr Emerg Care. 2012;28:606–610. , , , , .
- Healthcare Cost and Utilization Project. Cost‐to‐charge ratio files. Available at: http://www.hcup‐us.ahrq.gov/db/state/costtocharge.jsp. Accessed September 11, 2014.
- Risk of serious bacterial infection in young febrile infants with respiratory syncytial virus infections. Pediatrics. 2004;113:1728–1734. , , , et al.
- Establishing benchmarks for the hospitalized care of children with asthma, bronchiolitis, and pneumonia. Pediatrics. 2014;134:555–562. , , , et al.
- Well appearing young infants with fever without known source in the emergency department: are lumbar punctures always necessary? Eur J Emerg Med. 2010;17:167–169. , , , , , .
- Unpredictability of serious bacterial illness in febrile infants from birth to 1 month of age. Arch Pediatr Adolesc Med. 1999;153:508–511. , .
- Management and outcomes of care of fever in early infancy. JAMA. 2004;291:1203–1212. , , , et al.
- Performance of low‐risk criteria in the evaluation of young infants with fever: review of the literature. Pediatrics. 2010;125:228–233. , , .
- A week‐by‐week analysis of the low‐risk criteria for serious bacterial infection in febrile neonates. Arch Dis Child. 2009;94:287–292. , , , , , .
- Is 15 days an appropriate cut‐off age for considering serious bacterial infection in the management of febrile infants? Pediatr Infect Dis J. 2012;31:455–458. , , , et al.
- Outpatient management without antibiotics of fever in selected infants. N Engl J Med. 1993;329:1437–1441. , , .
- Identifying febrile infants at risk for a serious bacterial infection. J Pediatr. 1993;123:489–490. , , .
- Febrile infants at low risk for serious bacterial infection—an appraisal of the Rochester criteria and implications for management. Febrile Infant Collaborative Study Group. Pediatrics. 1994;94:390–396. , , , et al.
- American College of Emergency Physicians Clinical Policies Committee; American College of Emergency Physicians Clinical Policies Subcommittee on Pediatric Fever. Clinical policy for children younger than three years presenting to the emergency department with fever. Ann Emerg Med. 2003;42:530–545.
- Management of febrile neonates in US pediatric emergency departments. Pediatrics. 2014;133:187–195. , , , et al.
- Variation in care of the febrile young infant <90 days in US pediatric emergency departments. Pediatrics. 2014;134:667–677. , , , et al.
- Fever survey highlights significant variations in how infants aged ≤60 days are evaluated and underline the need for guidelines. Acta Paediatr. 2014;103:379–385. , , , , .
- Evidence‐based guidelines and critical pathways for quality improvement. Pediatrics. 1999;103:225–232. .
- Costs and infant outcomes after implementation of a care process model for febrile infants. Pediatrics. 2012;130:e16–e24. , , , et al.
- Research electronic data capture (REDCap)—a metadata‐driven methodology and workflow process for providing translational research informatics support. J Biomed Inform. 2009;42:377–381. , , , , , .
- Variation in occult injury screening for children with suspected abuse in selected US children's hospitals. Pediatrics. 2012;130:853–860. , , , , , .
- Achieving data quality. How data from a pediatric health information system earns the trust of its users. J AHIMA. 2004;75:22–26. .
- Corticosteroids and mortality in children with bacterial meningitis. JAMA. 2008;299:2048–2055. , , , .
- Variation in resource utilization across a national sample of pediatric emergency departments. J Pediatr. 2013;163:230–236. , , , et al.
- Deaths attributed to pediatric complex chronic conditions: national trends and implications for supportive care services. Pediatrics. 2001;107:E99. , , , , , .
- US Census Bureau. Geographic terms and concepts—census divisions and census regions. Available at: https://www.census.gov/geo/reference/gtc/gtc_census_divreg.html. Accessed September 10, 2014.
- Pediatric observation status: are we overlooking a growing population in children's hospitals? J Hosp Med. 2012;7:530–536. , , , et al.
- Differences in designations of observation care in US freestanding children's hospitals: are they virtual or real? J Hosp Med. 2012;7:287–293. , , , et al.
- Accuracy of administrative billing codes to detect urinary tract infection hospitalizations. Pediatrics. 2011;128:323–330. , , , et al.
- Identifying pediatric community‐acquired pneumonia hospitalizations: accuracy of administrative billing codes. JAMA Pediatr. 2013;167:851–858. , , , et al.
- Initial emergency department diagnosis and return visits: risk versus perception. Ann Emerg Med. 1998;32:569–573. , , , .
- A national depiction of children with return visits to the emergency department within 72 hours, 2001–2007. Pediatr Emerg Care. 2012;28:606–610. , , , , .
- Healthcare Cost and Utilization Project. Cost‐to‐charge ratio files. Available at: http://www.hcup‐us.ahrq.gov/db/state/costtocharge.jsp. Accessed September 11, 2014.
- Risk of serious bacterial infection in young febrile infants with respiratory syncytial virus infections. Pediatrics. 2004;113:1728–1734. , , , et al.
- Establishing benchmarks for the hospitalized care of children with asthma, bronchiolitis, and pneumonia. Pediatrics. 2014;134:555–562. , , , et al.
- Well appearing young infants with fever without known source in the emergency department: are lumbar punctures always necessary? Eur J Emerg Med. 2010;17:167–169. , , , , , .
- Unpredictability of serious bacterial illness in febrile infants from birth to 1 month of age. Arch Pediatr Adolesc Med. 1999;153:508–511. , .
- Management and outcomes of care of fever in early infancy. JAMA. 2004;291:1203–1212. , , , et al.
© 2015 Society of Hospital Medicine
Impact of In‐Hospital EVPCR Testing
Non‐polio enteroviruses are the most common cause of aseptic meningitis in children.1 While bacterial meningitis requires parenteral antibiotics, aseptic meningitis requires only supportive care.1 Enteroviral reverse transcription polymerase chain reaction (EVPCR) testing of the cerebrospinal fluid (CSF) allows the virus to be detected with high sensitivity and specificity.2 Because children with a positive EVPCR test are at low risk of bacterial meningitis,3 access to rapid EVPCR results has the potential to impact the clinical management of children with meningitis.4, 5 We studied the impact of implementing an in‐hospital EVPCR testing protocol on the clinical management of children with meningitis in a single‐center retrospective cohort.
MATERIALS AND METHODS
Study Design and Population
We identified children, <19 years of age, with meningitis evaluated at a single tertiary care pediatric center between July 2006 and June 2010. We defined meningitis as a CSF white blood cell (WBC) count 10 cells/mm3 corrected for the presence of CSF red blood cells (RBCs) (1 WBC for every 500 RBCs).6 We excluded children with any of the following: critical illness (defined as hypotension or respiratory failure), purpura, recent neurosurgery, ventricular shunt, immunosuppression, focal bacterial infection requiring parenteral antibiotics, positive CSF Gram stain, or known Lyme disease. The Institutional Review Board approved this study with waiver of informed consent.
Data Collection and Case Definitions
We abstracted historical and physical examination findings, as well as laboratory and microbiologic results, from the medical record. We defined bacterial meningitis as the isolation of pathogenic bacteria from the CSF or blood cultures. Children who had received antibiotics within 72 hours of diagnostic lumbar puncture, with negative cultures, were considered to have pretreated culture‐negative meningitis. Otherwise, children with negative bacterial cultures were classified as having aseptic meningitis.
EVPCR Testing
During the study pre‐period (July 1, 2006 through June 23, 2008), EVPCR tests were flown once daily to a commercial laboratory (ARUP Laboratories, Salt Lake City, UT) where they were run in batches. During the post‐period (June 24, 2008 through June 30, 2010), the study institution replaced the send‐out test with an in‐hospital EVPCR test (Gene Xpert EV Technology; Cepheid, Sunnyvale, CA)7 that allows multiple specimens to be run simultaneously, multiple times daily (between 7:00 AM and 10:00 PM), with results available in as little as 2.5 hours. We defined turnaround time for the test from specimen obtainment to test result.
Outcome Measures
Our 2 primary outcomes were length of stay and duration of parenteral antibiotics. Length of stay was measured as time from emergency department arrival to discharge (emergency department or inpatient discharge). We defined the duration of parenteral antibiotics as time from the first to the last dose of parenteral antibiotics administered, plus the standard antibiotic dosing interval for that antibiotic. For children with Lyme meningitis, the duration of parenteral antibiotic coverage was defined a priori as 48 hours, the standard time to reliably exclude bacterial growth from culture.8
Statistical Methods
Primary outcomes were compared using univariate analyses in 6 patient groups: 1) all patients, and those with 2) a positive EVPCR test, 3) a negative EVPCR test, and a positive test who were 4) 90 days old, 5) >90 days old, and 6) presented during peak enteroviral season (June through October). We utilized MannWhitney tests for continuous variables and 2 tests for proportions. We compared the median turnaround time for EVPCR results and the percentage of tests returning prior to discharge between the pre‐ and post‐periods. We performed interrupted time series spline analyses to assess for trends in our primary outcomes, independent of the change in EVPCR testing protocol. All analyses were conducted using the Statistical Package for the Social Sciences (IBM SPSS Inc, Chicago, IL).9
RESULTS
Of the 593 children with meningitis, 152 (26%) were excluded for the reasons noted above. The 441 patients included in our analyses had the following final diagnoses: bacterial meningitis (1 patient with Streptococcus pneumoniae, 0.2%), pretreated culture‐negative meningitis (42 patients, 10%), and aseptic meningitis (398 patients, 90%).
We compared patient populations and EVPCR testing characteristics between the pre‐ and post‐study periods (Table 1). While CSF glucose differed between study periods, the difference was not felt to be clinically significant. However, during the post‐period, more children presented during enteroviral season. Clinicians were more likely to order an EVPCR test for children with aseptic, than bacterial, meningitis (213/370 [58%] vs 0/1 [0%]).
Characteristic | Pre‐period (N = 225) | Post‐period (N = 216) | P Value |
---|---|---|---|
| |||
Demographics | |||
Age (months)* | 3 (2106) | 3 (188) | 0.20 |
Male, n (%) | 135 (60) | 129 (60) | 0.95 |
Historical features | |||
Duration of illness (days)* | 2 (14) | 2 (14) | 0.20 |
Duration of fever (days)* | 1 (12) | 1 (12) | 0.52 |
Antibiotic pretreatment, n (%) | 29 (13) | 13 (6.0) | 0.015 |
Temperature at ED presentation* (C) | 37.6 (36.838.4) | 37.8 (37.138.2) | 0.51 |
Presentation June through October, n (%) | 127 (56) | 143 (66) | 0.040 |
Laboratory results | |||
Peripheral WBC (cells/mm3)* | 10.4 (8.213.7) | 10.4 (7.813.6) | 0.67 |
Peripheral ANC (cells/mm3)* | 5.2 (3.17.4) | 4.9 (2.68.2) | 0.47 |
CSF WBC (cells/mm3)* | 55 (19176) | 62 (17250) | 0.66 |
CSF ANC (cells/mm3)* | 8 (045) | 7 (141) | 0.78 |
CSF glucose (mg/dL)* | 57 (5065) | 54 (4860) | 0.01 |
CSF protein(mg/dL)* | 50 (3480) | 48 (3470) | 0.73 |
Traumatic lumbar puncture (CSF RBC 500 cells/mm3), n (%) | 48 (21) | 43 (20) | 0.71 |
Patient management | |||
Admission to the hospital, n (%) | 196 (87) | 190 (88) | 0.68 |
Parenteral antibiotics initiated, n (%) | 206 (92) | 200 (93) | 0.80 |
Enteroviral PCR Testing | |||
Testing utilization, n (%) | 62 (28) | 133 (62) | <0.001 |
90 days of age, n (%) | 18 (16) | 57/114 (50) | <0.001 |
>90 days of age, n (%) | 44 (39) | 76/102 (75) | <0.001 |
Positive test result, n (%) | 33 (53) | 80 (60) | 0.22 |
Test turnaround time, hours* | 53 (4667) | 12 (617) | <0.001 |
We evaluated the impact of the in‐hospital EVPCR test on the length of stay and duration of parenteral antibiotics for the 6 predefined patient groups (Table 2). Length of stay could be determined for 432 (98%) of study patients, and duration of parenteral antibiotics for 365 (83%). We found a clinically important decrease in both length of stay and duration of parenteral antibiotics for children with a positive EVPCR test in the post‐period. For every hour earlier the EVPCR results returned, length of stay was reduced by 0.3 hours ( = 0.3, 95% confidence interval [CI] 0.10.5), and parenteral antibiotics were reduced by 0.3 hours ( = 0.3, 95% CI 0.10.5). However, even in the post‐period, the median length of time from a positive EVPCR test result to hospital discharge was 14 hours (interquartile range, 533 hours).
Patient Group | Pre‐Period | Post‐Period | P Value1 |
---|---|---|---|
| |||
1) All study patients | N = 225 | N = 216 | |
Length of stay* | 49 (2662) | 47 (2662) | 0.09 |
Duration of parenteral antibiotics* | 48 (2464) | 48 (2460) | 0.23 |
2) Children with a positive EVPCR test | N = 32 | N = 80 | |
Length of stay* | 44 (2854) | 28 (1946) | 0.005 |
Duration of parenteral antibiotics* | 48 (3072) | 36 (2449) | 0.037 |
3) Children with a negative EVPCR test | N = 29 | N = 53 | |
Length of stay* | 61 (30114) | 59 (45109) | 0.67 |
Duration of parenteral antibiotics* | 52 (4784) | 54 (4870) | 0.93 |
4) Children 90 days of age with positive EVPCR test | N = 9 | N =39 | |
Length of stay* | 66 (5071) | 37 (2753) | 0.003 |
Duration of parenteral antibiotics* | 74 (6994) | 48 (3660) | 0.002 |
5) Children >90 days of age with positive EVPCR test | N = 23 | N = 41 | |
Length of stay* | 32 (2750) | 21 (430) | 0.002 |
Duration of parenteral antibiotics* | 38 (2460) | 24 (2436) | 0.009 |
6) Children with a positive EVPCR test who presented during peak enteroviral season | N = 29 | N = 72 | |
Length of stay* | 43 (2853) | 26 (1738) | 0.002 |
Duration of parenteral antibiotics* | 46 (2470) | 36 (2448) | 0.05 |
We observed no trend in length of stay in either testing period ( = 0.17, 95% CI 3.9 to 3.6 pre vs = 1.64, 95% CI 6.3 to 3.0 post), with no change following the introduction of the faster EVPCR protocol (P = 0.52). We observed an increase in duration of parenteral antibiotics in the pre‐period ( = 5.4, 95% CI 0.3 to 10.6), with no trend in the post‐period ( = 1.7, 95% CI 5.2 to 1.8), but the difference was not significant (P = 0.08).
DISCUSSION
The in‐hospital EVPCR testing protocol reduced test turnaround time and increased testing. Children with a positive test had a shorter length of stay and duration of parenteral antibiotics. Decreasing the test turnaround time for EVPCR improved the care of children with enteroviral meningitis by reducing the length of unnecessary hospitalizations and parenteral antibiotics, with the potential for reducing the costs associated with these admissions.
Accurate identification of children with enteroviral meningitis, an often self‐limited infection requiring supportive care, can reduce hospitalization and unnecessary antibiotics. Previously, a positive EVPCR test result has been associated with a reduction in length of stay and of parenteral antibiotics,4, 5, 1012 with a direct correlation between test turnaround time and length of stay.12, 13 Moreover, positive EVPCR test results that were available prior to hospital discharge resulted in shorter length of hospital stay and duration of parenteral antibiotics.10
Our study is the largest to investigate the impact of implementing an in‐hospital EVPCR testing protocol, with the goal of making results available in a clinically useful time frame for all patients. Older EVPCR tests were typically performed in batches, or at centralized laboratories.4, 5, 1013 The in‐hospital EVPCR test utilized is a simple testing platform, which can be run multiple times daily. While there were higher charges associated with increased testing in the post‐period, these were more than offset by a reduced length of stay. Using study institution patient charges, we estimate that overall patient charges decreased approximately $80,000 in the post‐period, compared to the pre‐period (an average reduction of $375 per patient).
Many children were not discharged when a positive EVPCR test result became available. Some children with enteroviral meningitis will have persistent symptoms that require inpatient management. In addition, results that returned in the evening or nighttime were less likely to result in immediate hospital discharge. However, children with a positive EVPCR test are at very low risk for bacterial meningitis.3 As clinicians' knowledge of, and comfort with, the EVPCR test increase, this technology has the potential to further decrease the costs of caring for children with enteroviral meningitis.14
Our study had several limitations. First, it was retrospective; however, primary outcomes were objective measures accurately recorded in the medical record for most patients. Second, our study was single‐center, and findings may not be generalizable to other settings. Third, the management of children with meningitis may have been changing over the study period, independent of the in‐hospital EVPCR test. However, among children with a negative test, we observed no change in either of our primary outcomes. Fourth, given the large number of physicians involved with testing and treatment decisions, we could not adjust for clustering at the physician level. Fifth, we corrected CSF WBC in the case of a traumatic lumbar puncture (LP). Although use of this correction might underestimate the true CSF WBC count,6 the percentage of children with traumatic lumbar punctures was the same in both testing periods. Lastly, we evaluated the impact of a diagnostic test immediately after introduction into the clinical setting. As new medical technologies often take time to be adopted into clinical practice,15 we would expect the impact to increase over time.
CONCLUSIONS
In‐hospital EVPCR testing can improve the care of children with meningitis by reducing the length of unnecessary hospitalizations and parenteral antibiotics. Clinicians caring for children with meningitis should have access to in‐hospital EVPCR testing.
Acknowledgements
Disclosure: Nothing to report.
- Enteroviral infections of the central nervous system.Clin Infect Dis.1995;20(4):971–981. .
- Clinical utility of the polymerase chain reaction for diagnosis of enteroviral meningitis in infancy.J Pediatr.1997;131(3):393–397. , , , et al.
- Low risk of bacterial meningitis in children with a positive enteroviral polymerase chain reaction test result.Clin Infect Dis.2010;51(10):1221–1222. , , , .
- Impact of rapid polymerase chain reaction results on management of pediatric patients with enteroviral meningitis.Pediatr Infect Dis J.2002;21(4):283–286. , , , , , .
- Routine cerebrospinal fluid enterovirus polymerase chain reaction testing reduces hospitalization and antibiotic use for infants 90 days of age or younger.Pediatrics.2007;120(3):489–496. , , , , , .
- Traumatic lumbar punctures in neonates: test performance of the cerebrospinal fluid white blood cell count.Pediatr Infect Dis J.2008;27(12):1047–1051. , , , , , .
- Multicenter beta trial of the GeneXpert enterovirus assay.J Clin Microbiol.2007;45(4):1081–1086. , , , et al.
- Most cerebrospinal fluid cultures in children with bacterial meningitis are positive within two days.Pediatr Infect Dis J.1999;18(8):732–733. , .
- SPSS for Windows [computer program]. Version 19.0.0.Chicago, IL:IBM SPSS Inc;2009.
- Impact of a diagnostic cerebrospinal fluid enterovirus polymerase chain reaction test on patient management.JAMA.2000;283(20):2680–2685. , , , , .
- The impact of an enteroviral RT‐PCR assay on the diagnosis of aseptic meningitis and patient management.J Clin Virol.2002;25(suppl 1):S19–S26. , , , , .
- Impact of rapid enterovirus molecular diagnosis on the management of infants, children, and adults with aseptic meningitis.J Med Virol.2009;81(1):42–48. , , , et al.
- A one‐step RT‐PCR assay using an enzyme‐linked detection system for the diagnosis of enterovirus meningitis.J Clin Virol.2000;17(3):143–149. , , , et al.
- Cost analysis of enteroviral polymerase chain reaction in infants with fever and cerebrospinal fluid pleocytosis.Arch Pediatr Adolesc Med.2000;154(8):817–821. , .
- Adoption of new surgical technology.BMJ.2006;332(7533):112–114. .
Non‐polio enteroviruses are the most common cause of aseptic meningitis in children.1 While bacterial meningitis requires parenteral antibiotics, aseptic meningitis requires only supportive care.1 Enteroviral reverse transcription polymerase chain reaction (EVPCR) testing of the cerebrospinal fluid (CSF) allows the virus to be detected with high sensitivity and specificity.2 Because children with a positive EVPCR test are at low risk of bacterial meningitis,3 access to rapid EVPCR results has the potential to impact the clinical management of children with meningitis.4, 5 We studied the impact of implementing an in‐hospital EVPCR testing protocol on the clinical management of children with meningitis in a single‐center retrospective cohort.
MATERIALS AND METHODS
Study Design and Population
We identified children, <19 years of age, with meningitis evaluated at a single tertiary care pediatric center between July 2006 and June 2010. We defined meningitis as a CSF white blood cell (WBC) count 10 cells/mm3 corrected for the presence of CSF red blood cells (RBCs) (1 WBC for every 500 RBCs).6 We excluded children with any of the following: critical illness (defined as hypotension or respiratory failure), purpura, recent neurosurgery, ventricular shunt, immunosuppression, focal bacterial infection requiring parenteral antibiotics, positive CSF Gram stain, or known Lyme disease. The Institutional Review Board approved this study with waiver of informed consent.
Data Collection and Case Definitions
We abstracted historical and physical examination findings, as well as laboratory and microbiologic results, from the medical record. We defined bacterial meningitis as the isolation of pathogenic bacteria from the CSF or blood cultures. Children who had received antibiotics within 72 hours of diagnostic lumbar puncture, with negative cultures, were considered to have pretreated culture‐negative meningitis. Otherwise, children with negative bacterial cultures were classified as having aseptic meningitis.
EVPCR Testing
During the study pre‐period (July 1, 2006 through June 23, 2008), EVPCR tests were flown once daily to a commercial laboratory (ARUP Laboratories, Salt Lake City, UT) where they were run in batches. During the post‐period (June 24, 2008 through June 30, 2010), the study institution replaced the send‐out test with an in‐hospital EVPCR test (Gene Xpert EV Technology; Cepheid, Sunnyvale, CA)7 that allows multiple specimens to be run simultaneously, multiple times daily (between 7:00 AM and 10:00 PM), with results available in as little as 2.5 hours. We defined turnaround time for the test from specimen obtainment to test result.
Outcome Measures
Our 2 primary outcomes were length of stay and duration of parenteral antibiotics. Length of stay was measured as time from emergency department arrival to discharge (emergency department or inpatient discharge). We defined the duration of parenteral antibiotics as time from the first to the last dose of parenteral antibiotics administered, plus the standard antibiotic dosing interval for that antibiotic. For children with Lyme meningitis, the duration of parenteral antibiotic coverage was defined a priori as 48 hours, the standard time to reliably exclude bacterial growth from culture.8
Statistical Methods
Primary outcomes were compared using univariate analyses in 6 patient groups: 1) all patients, and those with 2) a positive EVPCR test, 3) a negative EVPCR test, and a positive test who were 4) 90 days old, 5) >90 days old, and 6) presented during peak enteroviral season (June through October). We utilized MannWhitney tests for continuous variables and 2 tests for proportions. We compared the median turnaround time for EVPCR results and the percentage of tests returning prior to discharge between the pre‐ and post‐periods. We performed interrupted time series spline analyses to assess for trends in our primary outcomes, independent of the change in EVPCR testing protocol. All analyses were conducted using the Statistical Package for the Social Sciences (IBM SPSS Inc, Chicago, IL).9
RESULTS
Of the 593 children with meningitis, 152 (26%) were excluded for the reasons noted above. The 441 patients included in our analyses had the following final diagnoses: bacterial meningitis (1 patient with Streptococcus pneumoniae, 0.2%), pretreated culture‐negative meningitis (42 patients, 10%), and aseptic meningitis (398 patients, 90%).
We compared patient populations and EVPCR testing characteristics between the pre‐ and post‐study periods (Table 1). While CSF glucose differed between study periods, the difference was not felt to be clinically significant. However, during the post‐period, more children presented during enteroviral season. Clinicians were more likely to order an EVPCR test for children with aseptic, than bacterial, meningitis (213/370 [58%] vs 0/1 [0%]).
Characteristic | Pre‐period (N = 225) | Post‐period (N = 216) | P Value |
---|---|---|---|
| |||
Demographics | |||
Age (months)* | 3 (2106) | 3 (188) | 0.20 |
Male, n (%) | 135 (60) | 129 (60) | 0.95 |
Historical features | |||
Duration of illness (days)* | 2 (14) | 2 (14) | 0.20 |
Duration of fever (days)* | 1 (12) | 1 (12) | 0.52 |
Antibiotic pretreatment, n (%) | 29 (13) | 13 (6.0) | 0.015 |
Temperature at ED presentation* (C) | 37.6 (36.838.4) | 37.8 (37.138.2) | 0.51 |
Presentation June through October, n (%) | 127 (56) | 143 (66) | 0.040 |
Laboratory results | |||
Peripheral WBC (cells/mm3)* | 10.4 (8.213.7) | 10.4 (7.813.6) | 0.67 |
Peripheral ANC (cells/mm3)* | 5.2 (3.17.4) | 4.9 (2.68.2) | 0.47 |
CSF WBC (cells/mm3)* | 55 (19176) | 62 (17250) | 0.66 |
CSF ANC (cells/mm3)* | 8 (045) | 7 (141) | 0.78 |
CSF glucose (mg/dL)* | 57 (5065) | 54 (4860) | 0.01 |
CSF protein(mg/dL)* | 50 (3480) | 48 (3470) | 0.73 |
Traumatic lumbar puncture (CSF RBC 500 cells/mm3), n (%) | 48 (21) | 43 (20) | 0.71 |
Patient management | |||
Admission to the hospital, n (%) | 196 (87) | 190 (88) | 0.68 |
Parenteral antibiotics initiated, n (%) | 206 (92) | 200 (93) | 0.80 |
Enteroviral PCR Testing | |||
Testing utilization, n (%) | 62 (28) | 133 (62) | <0.001 |
90 days of age, n (%) | 18 (16) | 57/114 (50) | <0.001 |
>90 days of age, n (%) | 44 (39) | 76/102 (75) | <0.001 |
Positive test result, n (%) | 33 (53) | 80 (60) | 0.22 |
Test turnaround time, hours* | 53 (4667) | 12 (617) | <0.001 |
We evaluated the impact of the in‐hospital EVPCR test on the length of stay and duration of parenteral antibiotics for the 6 predefined patient groups (Table 2). Length of stay could be determined for 432 (98%) of study patients, and duration of parenteral antibiotics for 365 (83%). We found a clinically important decrease in both length of stay and duration of parenteral antibiotics for children with a positive EVPCR test in the post‐period. For every hour earlier the EVPCR results returned, length of stay was reduced by 0.3 hours ( = 0.3, 95% confidence interval [CI] 0.10.5), and parenteral antibiotics were reduced by 0.3 hours ( = 0.3, 95% CI 0.10.5). However, even in the post‐period, the median length of time from a positive EVPCR test result to hospital discharge was 14 hours (interquartile range, 533 hours).
Patient Group | Pre‐Period | Post‐Period | P Value1 |
---|---|---|---|
| |||
1) All study patients | N = 225 | N = 216 | |
Length of stay* | 49 (2662) | 47 (2662) | 0.09 |
Duration of parenteral antibiotics* | 48 (2464) | 48 (2460) | 0.23 |
2) Children with a positive EVPCR test | N = 32 | N = 80 | |
Length of stay* | 44 (2854) | 28 (1946) | 0.005 |
Duration of parenteral antibiotics* | 48 (3072) | 36 (2449) | 0.037 |
3) Children with a negative EVPCR test | N = 29 | N = 53 | |
Length of stay* | 61 (30114) | 59 (45109) | 0.67 |
Duration of parenteral antibiotics* | 52 (4784) | 54 (4870) | 0.93 |
4) Children 90 days of age with positive EVPCR test | N = 9 | N =39 | |
Length of stay* | 66 (5071) | 37 (2753) | 0.003 |
Duration of parenteral antibiotics* | 74 (6994) | 48 (3660) | 0.002 |
5) Children >90 days of age with positive EVPCR test | N = 23 | N = 41 | |
Length of stay* | 32 (2750) | 21 (430) | 0.002 |
Duration of parenteral antibiotics* | 38 (2460) | 24 (2436) | 0.009 |
6) Children with a positive EVPCR test who presented during peak enteroviral season | N = 29 | N = 72 | |
Length of stay* | 43 (2853) | 26 (1738) | 0.002 |
Duration of parenteral antibiotics* | 46 (2470) | 36 (2448) | 0.05 |
We observed no trend in length of stay in either testing period ( = 0.17, 95% CI 3.9 to 3.6 pre vs = 1.64, 95% CI 6.3 to 3.0 post), with no change following the introduction of the faster EVPCR protocol (P = 0.52). We observed an increase in duration of parenteral antibiotics in the pre‐period ( = 5.4, 95% CI 0.3 to 10.6), with no trend in the post‐period ( = 1.7, 95% CI 5.2 to 1.8), but the difference was not significant (P = 0.08).
DISCUSSION
The in‐hospital EVPCR testing protocol reduced test turnaround time and increased testing. Children with a positive test had a shorter length of stay and duration of parenteral antibiotics. Decreasing the test turnaround time for EVPCR improved the care of children with enteroviral meningitis by reducing the length of unnecessary hospitalizations and parenteral antibiotics, with the potential for reducing the costs associated with these admissions.
Accurate identification of children with enteroviral meningitis, an often self‐limited infection requiring supportive care, can reduce hospitalization and unnecessary antibiotics. Previously, a positive EVPCR test result has been associated with a reduction in length of stay and of parenteral antibiotics,4, 5, 1012 with a direct correlation between test turnaround time and length of stay.12, 13 Moreover, positive EVPCR test results that were available prior to hospital discharge resulted in shorter length of hospital stay and duration of parenteral antibiotics.10
Our study is the largest to investigate the impact of implementing an in‐hospital EVPCR testing protocol, with the goal of making results available in a clinically useful time frame for all patients. Older EVPCR tests were typically performed in batches, or at centralized laboratories.4, 5, 1013 The in‐hospital EVPCR test utilized is a simple testing platform, which can be run multiple times daily. While there were higher charges associated with increased testing in the post‐period, these were more than offset by a reduced length of stay. Using study institution patient charges, we estimate that overall patient charges decreased approximately $80,000 in the post‐period, compared to the pre‐period (an average reduction of $375 per patient).
Many children were not discharged when a positive EVPCR test result became available. Some children with enteroviral meningitis will have persistent symptoms that require inpatient management. In addition, results that returned in the evening or nighttime were less likely to result in immediate hospital discharge. However, children with a positive EVPCR test are at very low risk for bacterial meningitis.3 As clinicians' knowledge of, and comfort with, the EVPCR test increase, this technology has the potential to further decrease the costs of caring for children with enteroviral meningitis.14
Our study had several limitations. First, it was retrospective; however, primary outcomes were objective measures accurately recorded in the medical record for most patients. Second, our study was single‐center, and findings may not be generalizable to other settings. Third, the management of children with meningitis may have been changing over the study period, independent of the in‐hospital EVPCR test. However, among children with a negative test, we observed no change in either of our primary outcomes. Fourth, given the large number of physicians involved with testing and treatment decisions, we could not adjust for clustering at the physician level. Fifth, we corrected CSF WBC in the case of a traumatic lumbar puncture (LP). Although use of this correction might underestimate the true CSF WBC count,6 the percentage of children with traumatic lumbar punctures was the same in both testing periods. Lastly, we evaluated the impact of a diagnostic test immediately after introduction into the clinical setting. As new medical technologies often take time to be adopted into clinical practice,15 we would expect the impact to increase over time.
CONCLUSIONS
In‐hospital EVPCR testing can improve the care of children with meningitis by reducing the length of unnecessary hospitalizations and parenteral antibiotics. Clinicians caring for children with meningitis should have access to in‐hospital EVPCR testing.
Acknowledgements
Disclosure: Nothing to report.
Non‐polio enteroviruses are the most common cause of aseptic meningitis in children.1 While bacterial meningitis requires parenteral antibiotics, aseptic meningitis requires only supportive care.1 Enteroviral reverse transcription polymerase chain reaction (EVPCR) testing of the cerebrospinal fluid (CSF) allows the virus to be detected with high sensitivity and specificity.2 Because children with a positive EVPCR test are at low risk of bacterial meningitis,3 access to rapid EVPCR results has the potential to impact the clinical management of children with meningitis.4, 5 We studied the impact of implementing an in‐hospital EVPCR testing protocol on the clinical management of children with meningitis in a single‐center retrospective cohort.
MATERIALS AND METHODS
Study Design and Population
We identified children, <19 years of age, with meningitis evaluated at a single tertiary care pediatric center between July 2006 and June 2010. We defined meningitis as a CSF white blood cell (WBC) count 10 cells/mm3 corrected for the presence of CSF red blood cells (RBCs) (1 WBC for every 500 RBCs).6 We excluded children with any of the following: critical illness (defined as hypotension or respiratory failure), purpura, recent neurosurgery, ventricular shunt, immunosuppression, focal bacterial infection requiring parenteral antibiotics, positive CSF Gram stain, or known Lyme disease. The Institutional Review Board approved this study with waiver of informed consent.
Data Collection and Case Definitions
We abstracted historical and physical examination findings, as well as laboratory and microbiologic results, from the medical record. We defined bacterial meningitis as the isolation of pathogenic bacteria from the CSF or blood cultures. Children who had received antibiotics within 72 hours of diagnostic lumbar puncture, with negative cultures, were considered to have pretreated culture‐negative meningitis. Otherwise, children with negative bacterial cultures were classified as having aseptic meningitis.
EVPCR Testing
During the study pre‐period (July 1, 2006 through June 23, 2008), EVPCR tests were flown once daily to a commercial laboratory (ARUP Laboratories, Salt Lake City, UT) where they were run in batches. During the post‐period (June 24, 2008 through June 30, 2010), the study institution replaced the send‐out test with an in‐hospital EVPCR test (Gene Xpert EV Technology; Cepheid, Sunnyvale, CA)7 that allows multiple specimens to be run simultaneously, multiple times daily (between 7:00 AM and 10:00 PM), with results available in as little as 2.5 hours. We defined turnaround time for the test from specimen obtainment to test result.
Outcome Measures
Our 2 primary outcomes were length of stay and duration of parenteral antibiotics. Length of stay was measured as time from emergency department arrival to discharge (emergency department or inpatient discharge). We defined the duration of parenteral antibiotics as time from the first to the last dose of parenteral antibiotics administered, plus the standard antibiotic dosing interval for that antibiotic. For children with Lyme meningitis, the duration of parenteral antibiotic coverage was defined a priori as 48 hours, the standard time to reliably exclude bacterial growth from culture.8
Statistical Methods
Primary outcomes were compared using univariate analyses in 6 patient groups: 1) all patients, and those with 2) a positive EVPCR test, 3) a negative EVPCR test, and a positive test who were 4) 90 days old, 5) >90 days old, and 6) presented during peak enteroviral season (June through October). We utilized MannWhitney tests for continuous variables and 2 tests for proportions. We compared the median turnaround time for EVPCR results and the percentage of tests returning prior to discharge between the pre‐ and post‐periods. We performed interrupted time series spline analyses to assess for trends in our primary outcomes, independent of the change in EVPCR testing protocol. All analyses were conducted using the Statistical Package for the Social Sciences (IBM SPSS Inc, Chicago, IL).9
RESULTS
Of the 593 children with meningitis, 152 (26%) were excluded for the reasons noted above. The 441 patients included in our analyses had the following final diagnoses: bacterial meningitis (1 patient with Streptococcus pneumoniae, 0.2%), pretreated culture‐negative meningitis (42 patients, 10%), and aseptic meningitis (398 patients, 90%).
We compared patient populations and EVPCR testing characteristics between the pre‐ and post‐study periods (Table 1). While CSF glucose differed between study periods, the difference was not felt to be clinically significant. However, during the post‐period, more children presented during enteroviral season. Clinicians were more likely to order an EVPCR test for children with aseptic, than bacterial, meningitis (213/370 [58%] vs 0/1 [0%]).
Characteristic | Pre‐period (N = 225) | Post‐period (N = 216) | P Value |
---|---|---|---|
| |||
Demographics | |||
Age (months)* | 3 (2106) | 3 (188) | 0.20 |
Male, n (%) | 135 (60) | 129 (60) | 0.95 |
Historical features | |||
Duration of illness (days)* | 2 (14) | 2 (14) | 0.20 |
Duration of fever (days)* | 1 (12) | 1 (12) | 0.52 |
Antibiotic pretreatment, n (%) | 29 (13) | 13 (6.0) | 0.015 |
Temperature at ED presentation* (C) | 37.6 (36.838.4) | 37.8 (37.138.2) | 0.51 |
Presentation June through October, n (%) | 127 (56) | 143 (66) | 0.040 |
Laboratory results | |||
Peripheral WBC (cells/mm3)* | 10.4 (8.213.7) | 10.4 (7.813.6) | 0.67 |
Peripheral ANC (cells/mm3)* | 5.2 (3.17.4) | 4.9 (2.68.2) | 0.47 |
CSF WBC (cells/mm3)* | 55 (19176) | 62 (17250) | 0.66 |
CSF ANC (cells/mm3)* | 8 (045) | 7 (141) | 0.78 |
CSF glucose (mg/dL)* | 57 (5065) | 54 (4860) | 0.01 |
CSF protein(mg/dL)* | 50 (3480) | 48 (3470) | 0.73 |
Traumatic lumbar puncture (CSF RBC 500 cells/mm3), n (%) | 48 (21) | 43 (20) | 0.71 |
Patient management | |||
Admission to the hospital, n (%) | 196 (87) | 190 (88) | 0.68 |
Parenteral antibiotics initiated, n (%) | 206 (92) | 200 (93) | 0.80 |
Enteroviral PCR Testing | |||
Testing utilization, n (%) | 62 (28) | 133 (62) | <0.001 |
90 days of age, n (%) | 18 (16) | 57/114 (50) | <0.001 |
>90 days of age, n (%) | 44 (39) | 76/102 (75) | <0.001 |
Positive test result, n (%) | 33 (53) | 80 (60) | 0.22 |
Test turnaround time, hours* | 53 (4667) | 12 (617) | <0.001 |
We evaluated the impact of the in‐hospital EVPCR test on the length of stay and duration of parenteral antibiotics for the 6 predefined patient groups (Table 2). Length of stay could be determined for 432 (98%) of study patients, and duration of parenteral antibiotics for 365 (83%). We found a clinically important decrease in both length of stay and duration of parenteral antibiotics for children with a positive EVPCR test in the post‐period. For every hour earlier the EVPCR results returned, length of stay was reduced by 0.3 hours ( = 0.3, 95% confidence interval [CI] 0.10.5), and parenteral antibiotics were reduced by 0.3 hours ( = 0.3, 95% CI 0.10.5). However, even in the post‐period, the median length of time from a positive EVPCR test result to hospital discharge was 14 hours (interquartile range, 533 hours).
Patient Group | Pre‐Period | Post‐Period | P Value1 |
---|---|---|---|
| |||
1) All study patients | N = 225 | N = 216 | |
Length of stay* | 49 (2662) | 47 (2662) | 0.09 |
Duration of parenteral antibiotics* | 48 (2464) | 48 (2460) | 0.23 |
2) Children with a positive EVPCR test | N = 32 | N = 80 | |
Length of stay* | 44 (2854) | 28 (1946) | 0.005 |
Duration of parenteral antibiotics* | 48 (3072) | 36 (2449) | 0.037 |
3) Children with a negative EVPCR test | N = 29 | N = 53 | |
Length of stay* | 61 (30114) | 59 (45109) | 0.67 |
Duration of parenteral antibiotics* | 52 (4784) | 54 (4870) | 0.93 |
4) Children 90 days of age with positive EVPCR test | N = 9 | N =39 | |
Length of stay* | 66 (5071) | 37 (2753) | 0.003 |
Duration of parenteral antibiotics* | 74 (6994) | 48 (3660) | 0.002 |
5) Children >90 days of age with positive EVPCR test | N = 23 | N = 41 | |
Length of stay* | 32 (2750) | 21 (430) | 0.002 |
Duration of parenteral antibiotics* | 38 (2460) | 24 (2436) | 0.009 |
6) Children with a positive EVPCR test who presented during peak enteroviral season | N = 29 | N = 72 | |
Length of stay* | 43 (2853) | 26 (1738) | 0.002 |
Duration of parenteral antibiotics* | 46 (2470) | 36 (2448) | 0.05 |
We observed no trend in length of stay in either testing period ( = 0.17, 95% CI 3.9 to 3.6 pre vs = 1.64, 95% CI 6.3 to 3.0 post), with no change following the introduction of the faster EVPCR protocol (P = 0.52). We observed an increase in duration of parenteral antibiotics in the pre‐period ( = 5.4, 95% CI 0.3 to 10.6), with no trend in the post‐period ( = 1.7, 95% CI 5.2 to 1.8), but the difference was not significant (P = 0.08).
DISCUSSION
The in‐hospital EVPCR testing protocol reduced test turnaround time and increased testing. Children with a positive test had a shorter length of stay and duration of parenteral antibiotics. Decreasing the test turnaround time for EVPCR improved the care of children with enteroviral meningitis by reducing the length of unnecessary hospitalizations and parenteral antibiotics, with the potential for reducing the costs associated with these admissions.
Accurate identification of children with enteroviral meningitis, an often self‐limited infection requiring supportive care, can reduce hospitalization and unnecessary antibiotics. Previously, a positive EVPCR test result has been associated with a reduction in length of stay and of parenteral antibiotics,4, 5, 1012 with a direct correlation between test turnaround time and length of stay.12, 13 Moreover, positive EVPCR test results that were available prior to hospital discharge resulted in shorter length of hospital stay and duration of parenteral antibiotics.10
Our study is the largest to investigate the impact of implementing an in‐hospital EVPCR testing protocol, with the goal of making results available in a clinically useful time frame for all patients. Older EVPCR tests were typically performed in batches, or at centralized laboratories.4, 5, 1013 The in‐hospital EVPCR test utilized is a simple testing platform, which can be run multiple times daily. While there were higher charges associated with increased testing in the post‐period, these were more than offset by a reduced length of stay. Using study institution patient charges, we estimate that overall patient charges decreased approximately $80,000 in the post‐period, compared to the pre‐period (an average reduction of $375 per patient).
Many children were not discharged when a positive EVPCR test result became available. Some children with enteroviral meningitis will have persistent symptoms that require inpatient management. In addition, results that returned in the evening or nighttime were less likely to result in immediate hospital discharge. However, children with a positive EVPCR test are at very low risk for bacterial meningitis.3 As clinicians' knowledge of, and comfort with, the EVPCR test increase, this technology has the potential to further decrease the costs of caring for children with enteroviral meningitis.14
Our study had several limitations. First, it was retrospective; however, primary outcomes were objective measures accurately recorded in the medical record for most patients. Second, our study was single‐center, and findings may not be generalizable to other settings. Third, the management of children with meningitis may have been changing over the study period, independent of the in‐hospital EVPCR test. However, among children with a negative test, we observed no change in either of our primary outcomes. Fourth, given the large number of physicians involved with testing and treatment decisions, we could not adjust for clustering at the physician level. Fifth, we corrected CSF WBC in the case of a traumatic lumbar puncture (LP). Although use of this correction might underestimate the true CSF WBC count,6 the percentage of children with traumatic lumbar punctures was the same in both testing periods. Lastly, we evaluated the impact of a diagnostic test immediately after introduction into the clinical setting. As new medical technologies often take time to be adopted into clinical practice,15 we would expect the impact to increase over time.
CONCLUSIONS
In‐hospital EVPCR testing can improve the care of children with meningitis by reducing the length of unnecessary hospitalizations and parenteral antibiotics. Clinicians caring for children with meningitis should have access to in‐hospital EVPCR testing.
Acknowledgements
Disclosure: Nothing to report.
- Enteroviral infections of the central nervous system.Clin Infect Dis.1995;20(4):971–981. .
- Clinical utility of the polymerase chain reaction for diagnosis of enteroviral meningitis in infancy.J Pediatr.1997;131(3):393–397. , , , et al.
- Low risk of bacterial meningitis in children with a positive enteroviral polymerase chain reaction test result.Clin Infect Dis.2010;51(10):1221–1222. , , , .
- Impact of rapid polymerase chain reaction results on management of pediatric patients with enteroviral meningitis.Pediatr Infect Dis J.2002;21(4):283–286. , , , , , .
- Routine cerebrospinal fluid enterovirus polymerase chain reaction testing reduces hospitalization and antibiotic use for infants 90 days of age or younger.Pediatrics.2007;120(3):489–496. , , , , , .
- Traumatic lumbar punctures in neonates: test performance of the cerebrospinal fluid white blood cell count.Pediatr Infect Dis J.2008;27(12):1047–1051. , , , , , .
- Multicenter beta trial of the GeneXpert enterovirus assay.J Clin Microbiol.2007;45(4):1081–1086. , , , et al.
- Most cerebrospinal fluid cultures in children with bacterial meningitis are positive within two days.Pediatr Infect Dis J.1999;18(8):732–733. , .
- SPSS for Windows [computer program]. Version 19.0.0.Chicago, IL:IBM SPSS Inc;2009.
- Impact of a diagnostic cerebrospinal fluid enterovirus polymerase chain reaction test on patient management.JAMA.2000;283(20):2680–2685. , , , , .
- The impact of an enteroviral RT‐PCR assay on the diagnosis of aseptic meningitis and patient management.J Clin Virol.2002;25(suppl 1):S19–S26. , , , , .
- Impact of rapid enterovirus molecular diagnosis on the management of infants, children, and adults with aseptic meningitis.J Med Virol.2009;81(1):42–48. , , , et al.
- A one‐step RT‐PCR assay using an enzyme‐linked detection system for the diagnosis of enterovirus meningitis.J Clin Virol.2000;17(3):143–149. , , , et al.
- Cost analysis of enteroviral polymerase chain reaction in infants with fever and cerebrospinal fluid pleocytosis.Arch Pediatr Adolesc Med.2000;154(8):817–821. , .
- Adoption of new surgical technology.BMJ.2006;332(7533):112–114. .
- Enteroviral infections of the central nervous system.Clin Infect Dis.1995;20(4):971–981. .
- Clinical utility of the polymerase chain reaction for diagnosis of enteroviral meningitis in infancy.J Pediatr.1997;131(3):393–397. , , , et al.
- Low risk of bacterial meningitis in children with a positive enteroviral polymerase chain reaction test result.Clin Infect Dis.2010;51(10):1221–1222. , , , .
- Impact of rapid polymerase chain reaction results on management of pediatric patients with enteroviral meningitis.Pediatr Infect Dis J.2002;21(4):283–286. , , , , , .
- Routine cerebrospinal fluid enterovirus polymerase chain reaction testing reduces hospitalization and antibiotic use for infants 90 days of age or younger.Pediatrics.2007;120(3):489–496. , , , , , .
- Traumatic lumbar punctures in neonates: test performance of the cerebrospinal fluid white blood cell count.Pediatr Infect Dis J.2008;27(12):1047–1051. , , , , , .
- Multicenter beta trial of the GeneXpert enterovirus assay.J Clin Microbiol.2007;45(4):1081–1086. , , , et al.
- Most cerebrospinal fluid cultures in children with bacterial meningitis are positive within two days.Pediatr Infect Dis J.1999;18(8):732–733. , .
- SPSS for Windows [computer program]. Version 19.0.0.Chicago, IL:IBM SPSS Inc;2009.
- Impact of a diagnostic cerebrospinal fluid enterovirus polymerase chain reaction test on patient management.JAMA.2000;283(20):2680–2685. , , , , .
- The impact of an enteroviral RT‐PCR assay on the diagnosis of aseptic meningitis and patient management.J Clin Virol.2002;25(suppl 1):S19–S26. , , , , .
- Impact of rapid enterovirus molecular diagnosis on the management of infants, children, and adults with aseptic meningitis.J Med Virol.2009;81(1):42–48. , , , et al.
- A one‐step RT‐PCR assay using an enzyme‐linked detection system for the diagnosis of enterovirus meningitis.J Clin Virol.2000;17(3):143–149. , , , et al.
- Cost analysis of enteroviral polymerase chain reaction in infants with fever and cerebrospinal fluid pleocytosis.Arch Pediatr Adolesc Med.2000;154(8):817–821. , .
- Adoption of new surgical technology.BMJ.2006;332(7533):112–114. .
Correction of CSF Protein
Traumatic lumbar puncture (LP) occurs when peripheral blood is introduced into the cerebrospinal fluid (CSF) as a result of needle trauma, which causes bleeding into the subarachnoid space. Traumatic LPs occur in up to 30% of LPs performed in children.1, 2 In addition to affecting the CSF white blood cell count, the presence of CSF red blood cells (RBCs) is associated with higher CSF protein concentrations due to the higher protein concentration in plasma compared with CSF and to the release of protein from lysed red blood cells. CSF protein concentration has been used in clinical decision rules for the prediction of bacterial meningitis in children.3 Elevated protein levels are difficult to interpret in cases of traumatic LP, and a diagnosis of bacterial meningitis may be more difficult to exclude on the basis of CSF test results.4
The interpretation of CSF protein levels is further complicated in the youngest infants due to both the changing composition of the CSF as well as the higher rates of traumatic LPs.5 Therefore, studies establishing a correction factor, adjusting observed CSF protein levels for the presence of CSF RBCs, that included predominantly older children may not be generalizable to neonates and young infants.6 We sought to determine the relationship between CSF RBC count and CSF protein in infants 56 days of age who underwent LP in the emergency department (ED).
METHODS
Study Design, Setting, and Participants
This cross‐sectional study was performed at The Children's Hospital of Philadelphia (Philadelphia, PA), an urban, tertiary care children's hospital. The Committees for the Protection of Human Subjects approved this study with a waiver of informed consent.
Infants 56 days of age and younger were eligible for inclusion if they had an LP performed as part of their ED evaluation between January 1, 2005 and July 31, 2009. At The Children's Hospital of Philadelphia, infants 56 days and younger routinely receive LPs for evaluation of fever.79 Patients undergoing LP in the ED were identified using computerized order entry records as previously described.5, 10
We excluded patients with conditions known to elevate CSF protein, including: serious bacterial infection (bacterial meningitis, urinary tract infection, bacteremia, pneumonia, septic arthritis, and bacterial gastroenteritis),11 presence of a ventricular shunt, aseptic meningitis (positive CSF enteroviral polymerase chain reaction or CSF herpes simplex virus polymerase chain reaction), congenital infections (eg, syphilis), seizure prior to presentation, and elevated bilirubin (if serum bilirubin was obtained). Due to the fact that grossly bloody CSF samples are difficult to interpret, we excluded those with a CSF RBC count >150,000 cells/mm3, a cutoff representing the 99th percentile of CSF RBC values in the cohort after applying other exclusion criteria.
Study Definitions
Bacterial meningitis was defined as either the isolation of a known bacterial pathogen from the CSF or, in patients who received antibiotics prior to evaluation, the combination of CSF pleocytosis and bacteria reported on CSF Gram stain. Bacteremia was defined as the isolation of a known bacterial pathogen from blood cultures excluding commensal skin flora. Urinary tract infection was defined as growth of a single known pathogen meeting 1 of 3 criteria: (1) 1000 colony‐forming units per mL for urine cultures obtained by suprapubic aspiration, (2) 50,000 colony‐forming units per mL from a catheterized specimen, or (3) 10,000 colony‐forming units per mL from a catheterized specimen in association with a positive urinalysis.1214
Statistical Analysis
Data analysis was performed using STATA version 12 (Stata Corp, College Station, TX). Linear regression was used to determine the association between CSF RBC and CSF protein. We analyzed the following groups of children: 1) all eligible patients; 2) children 28 days versus children >28 days; 3) vaginal versus cesarean delivery; and 4) patients without CSF pleocytosis. In the primary subanalysis, CSF pleocytosis was defined as CSF white blood cells (WBCs) >19 cells/mm3 for infants 28 days of age and CSF WBCs >9 cells/mm3 for infants 29 days of age, using reference values established by Kestenbaum et al.10 Alternate definitions of CSF pleocytosis were also examined using reference values proposed by Byington et al15 (age 28 days, >18 cells/mm3; age >29 days, >8.5 cells/mm3) and Chadwick et al16(age 0‐7 days, >26 cells/mm3; age 8‐28 days, >9 cells/mm3; age 29‐49 days, >8 cells/mm3; and age 50‐56 days, >7 cells/mm3). We did not correct CSF WBCs for the RBC count because prior studies suggest that such correction factors do not provide any advantage over uncorrected values.17 Finally, linear regression analysis was repeated while including subjects with >150,000 RBC/mm3 to determine the effect of including those patients on the association of CSF RBC count and protein concentrations. Subjects with grossly bloody CSF specimens, defined a priori as a CSF RBC >1,000,000/mm3, were excluded from this subanalysis.
RESULTS
There were 1986 infants, 56 days of age or younger, who underwent LP in the ED during the study period. Patients were excluded for the following reasons: missing medical record number (n = 16); missing CSF WBC, CSF RBC, or CSF protein values (n = 290); conditions known to elevate CSF protein concentrations (n = 426, as follows: presence of a ventricular shunt device [n = 48], serious bacterial infection [n = 149], congenital infection [n = 2], positive CSF polymerase chain reaction [PCR] test for either enterovirus or herpes simplex virus [n = 97], seizure prior to presentation [n = 98], or elevated serum bilirubin [n = 32]). An additional 13 patients with a CSF RBC count >150,000 cells/mm3 were also excluded.
For the remaining 1241 study infants, the median age was 34 days (interquartile range: 19 days‐46 days) and 554 patients (45%) were male. The median CSF RBC count was 40 cells/mm3 (interquartile range: 2‐1080 cells/mm3); 11.8% of patients had a CSF RBC count >10,000 cells/mm3.
CSF protein increased linearly with increasing CSF RBCs (Figure 1). The increase in the CSF protein concentration of 1.9 mg/dL per 1000 CSF RBCs for all patients was similar between different age groups and delivery types (Table 1). Restricting analysis to those patients without pleocytosis also yielded comparable results; applying 2 other definitions of pleocytosis did not change the magnitude of the association (Table 1).
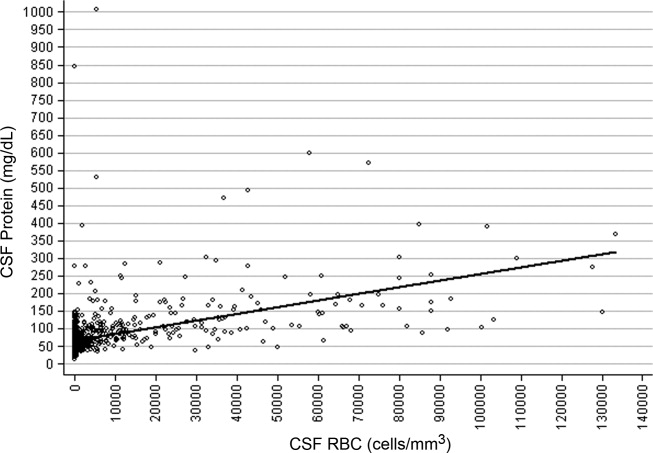
Patient Group | No. of Patients | Change in CSF protein (mg/dL) per 1000 RBCs (95% CI) |
---|---|---|
| ||
All eligible | 1241 | 1.9 (1.7‐2.1) |
No CSF pleocytosis* | 1085 | 2.0 (1.7‐2.4) |
Age | ||
Age 28 days | 481 | 1.9 (1.5‐2.3) |
Age >28 days | 760 | 1.9 (1.7‐2.1) |
Mode of delivery | ||
Vaginal | 741 | 1.9 (1.7‐2.2) |
Cesarean | 366 | 1.7 (1.4‐2.0) |
In a subanalysis, we then included subjects with a CSF RBC count >150,000/mm3; one extreme outlier with a CSF RBC equal to 3,160,000/mm3 remained excluded. Inclusion of more traumatic samples lessened the overall correction factor. The CSF protein increased by 1.22 mg/dL (95% confidence interval: 1.14‐1.29 mg/dL) per 1000 RBC/mm3 increase in the CSF. In the subset without CSF pleocytosis, the CSF protein increased by 1.44 mg/dL (95% confidence interval: 1.33‐1.57 mg/dL) per 1000 RBC/mm3.
Three children had high CSF protein values (>500 mg/dL) despite the relative paucity of CSF RBCs. Two of these infants had respiratory syncytial virus bronchiolitis; neither infant had signs or symptoms of neurological illness. While details of the labor and delivery were not available, the CSF sample for one of these infants was reported to have xanthochromia, and the other infant was reported to have had a traumatic LP with a CSF sample that subsequently cleared. The third infant had fever without a specific source identified, but had a birth history of vaginal delivery and prolonged labor. The CSF sample from LP for this patient was reported as grossly bloody by the performing clinicians and by the Clinical Microbiology Laboratory, despite a CSF red blood cell count of only 5500 cells/mm3.
DISCUSSION
In a large cohort of infants 56 days of age, CSF protein increased by approximately 2 mg/dL for every 1000 cell/mm3 increase in CSF RBCs. This correction factor is higher than previously reported correction factors from studies including older infants and children.6, 18 Some of this difference may be explained by the presence of old blood related to the trauma of labor and delivery. Previous work has demonstrated that the presence of xanthochromia, another RBC breakdown product, in the CSF of young infants was associated with maternal labor and elevated CSF protein.19 Consistent with this hypothesis, the correction factor was nominally higher in those infants born by vaginal delivery compared with those born by cesarean section.
Several infants in our study had high CSF protein levels despite a paucity of CSF RBCs. By convention at our institution, the protein and glucose values are determined from the second tube, and the WBCs and RBCs are determined from the third tube. However, we could not determine the order in which the specimens for protein and RBCs were collected for individual specimens. Additionally, it is possible that delayed clearance of blood from a traumatic LP would cause the CSF protein level to be high, as measured in the second tube, but lead to few RBCs in the third tube. These circumstances could explain the discrepancy between CSF protein and CSF RBCs counts for some patients.
The CSF protein adjustment factor for infants 56 days of age in our study was almost twice the correction of 1.1 mg/dL for every 1000 RBC increase reported by Nigrovic et al among infants 90 days of age.6 There are differences in the design of the 2 studies. We excluded subjects with exceedingly large numbers of CSF RBCs and restricted inclusion to those 56 days of age or younger. When subjects with >150,000 RBCs/mm3 were included, the correction decreased to a value comparable to that reported by Nigrovic et al.6 Therefore, it is possible that inclusion of subjects with grossly bloody specimens in prior studies skewed the association between CSF protein and CSF RBCs. The number of subjects in our cohort with >150,000 CSF RBCs was too small to calculate a relevant correction factor for infants with exceedingly high CSF RBC counts.
The results of this study should be considered in the context of several limitations. Details regarding labor and delivery were not available. We suspect that old blood related to the trauma of birth provides partial explanation for the higher correction factor in neonates and young infants compared with older children. However, differences in CSF blood‐brain barrier permeability may also contribute to these differences, independent of the CSF RBC count. Additionally, though the study population included a large number of neonates and young infants, a relatively small proportion of subjects had high CSF RBC counts. Therefore, our results may not be generalizable to those with exceedingly high CSF RBCs. Finally, available clinical prediction rules to identify patients with CSF pleocytosis, who are at very low risk for bacterial meningitis, include CSF protein as a predictor.3, 20, 21 Although CSF protein in children with traumatic LPs may need adjustment prior to application of the clinical prediction rule, further study is needed before implementing this approach.
In conclusion, we found that CSF protein concentrations increased by approximately 2 mg/dL for every 1000 CSF RBCs. Correction of CSF protein for those with extremely high CSF RBCs may not be appropriate, as conventional linear models do not apply. These data may assist clinicians in interpreting CSF protein concentrations in infants 56 days of age and younger in the context of traumatic LPs.
- Local anesthetic and stylet styles: factors associated with resident lumbar puncture success.Pediatrics.2006;117:876–881. , , , , , .
- Risk factors for traumatic or unsuccessful lumbar punctures in children.Ann Emerg Med.2007;49:762–771. , , .
- Clinical prediction rule for identifying children with cerebrospinal fluid pleocytosis at very low risk of bacterial meningitis.JAMA.2007;297:52–60. , , , et al.
- Interpretation of traumatic lumbar punctures: who can go home?Pediatrics.2003;111:525–528. , , .
- Age‐specific reference values for cerebrospinal fluid protein concentration in neonates and young infants.J Hosp Med.2011;6:22–27. , , , , .
- Correction of cerebrospinal fluid protein for the presence of red blood cells in children with a traumatic lumbar puncture.J Pediatr.2011;159:158–159. , , .
- Failure of infant observation scales in detecting serious illness in febrile, 4‐ to 8‐week‐old infants.Pediatrics.1990;85:1040–1043. , , .
- Unpredictability of serious bacterial illness in febrile infants from birth to 1 month of age.Arch Pediatr Adolesc Med.1999;153:508–511. , .
- Outpatient management without antibiotics of fever in selected infants.N Engl J Med.1993;329:1437–1441. , , .
- Defining cerebrospinal fluid white blood cell count reference values in neonates and young infants.Pediatrics.2010;125:257–264. , , , , .
- Sterile cerebrospinal fluid pleocytosis in young infants with urinary tract infections.J Pediatr.2008;153:290–292. , , , , .
- Clinical and demographic factors associated with urinary tract infection in young febrile infants.Pediatrics.2005;116:644–648. , , , et al.
- Prevalence of urinary tract infection in febrile young children in the emergency department.Pediatrics.1998;102:e16. , , , , .
- Prevalence of urinary tract infection in febrile infants.J Pediatr.1993;123:17–23. , , , , , .
- Normative cerebrospinal fluid profiles in febrile infants.J Pediatr.2011;158:130–134. , , .
- Cerebrospinal fluid characteristics of infants who present to the emergency department with fever: establishing normal values by week of age.Pediatr Infect Dis J.2011;30:e63–e67. , , , .
- Corrections for leukocytes and percent of neutrophils do not match observations in blood‐contaminated cerebrospinal fluid and have no value over uncorrected cells for diagnosis.Pediatr Infect Dis J.2006;25:8–11. , .
- Distinguishing cerebrospinal fluid abnormalities in children with bacterial meningitis and traumatic lumbar puncture.J Infect Dis.1990;162:251–254. , , , , .
- Cerebrospinal fluid xanthochromia in newborns is related to maternal labor before delivery.Pediatrics.2007;120:e1212–e1216. , , , .
- Accuracy and test characteristics of ancillary tests of cerebrospinal fluid for predicting acute bacterial meningitis in children with low white blood cell counts in cerebrospinal fluid.Acad Emerg Med.2005;12:303–309. , .
- A decision rule for predicting bacterial meningitis in children with cerebrospinal fluid pleocytosis when gram stain is negative or unavailable.Acad Emerg Med.2008;15:437–444. , , , .
Traumatic lumbar puncture (LP) occurs when peripheral blood is introduced into the cerebrospinal fluid (CSF) as a result of needle trauma, which causes bleeding into the subarachnoid space. Traumatic LPs occur in up to 30% of LPs performed in children.1, 2 In addition to affecting the CSF white blood cell count, the presence of CSF red blood cells (RBCs) is associated with higher CSF protein concentrations due to the higher protein concentration in plasma compared with CSF and to the release of protein from lysed red blood cells. CSF protein concentration has been used in clinical decision rules for the prediction of bacterial meningitis in children.3 Elevated protein levels are difficult to interpret in cases of traumatic LP, and a diagnosis of bacterial meningitis may be more difficult to exclude on the basis of CSF test results.4
The interpretation of CSF protein levels is further complicated in the youngest infants due to both the changing composition of the CSF as well as the higher rates of traumatic LPs.5 Therefore, studies establishing a correction factor, adjusting observed CSF protein levels for the presence of CSF RBCs, that included predominantly older children may not be generalizable to neonates and young infants.6 We sought to determine the relationship between CSF RBC count and CSF protein in infants 56 days of age who underwent LP in the emergency department (ED).
METHODS
Study Design, Setting, and Participants
This cross‐sectional study was performed at The Children's Hospital of Philadelphia (Philadelphia, PA), an urban, tertiary care children's hospital. The Committees for the Protection of Human Subjects approved this study with a waiver of informed consent.
Infants 56 days of age and younger were eligible for inclusion if they had an LP performed as part of their ED evaluation between January 1, 2005 and July 31, 2009. At The Children's Hospital of Philadelphia, infants 56 days and younger routinely receive LPs for evaluation of fever.79 Patients undergoing LP in the ED were identified using computerized order entry records as previously described.5, 10
We excluded patients with conditions known to elevate CSF protein, including: serious bacterial infection (bacterial meningitis, urinary tract infection, bacteremia, pneumonia, septic arthritis, and bacterial gastroenteritis),11 presence of a ventricular shunt, aseptic meningitis (positive CSF enteroviral polymerase chain reaction or CSF herpes simplex virus polymerase chain reaction), congenital infections (eg, syphilis), seizure prior to presentation, and elevated bilirubin (if serum bilirubin was obtained). Due to the fact that grossly bloody CSF samples are difficult to interpret, we excluded those with a CSF RBC count >150,000 cells/mm3, a cutoff representing the 99th percentile of CSF RBC values in the cohort after applying other exclusion criteria.
Study Definitions
Bacterial meningitis was defined as either the isolation of a known bacterial pathogen from the CSF or, in patients who received antibiotics prior to evaluation, the combination of CSF pleocytosis and bacteria reported on CSF Gram stain. Bacteremia was defined as the isolation of a known bacterial pathogen from blood cultures excluding commensal skin flora. Urinary tract infection was defined as growth of a single known pathogen meeting 1 of 3 criteria: (1) 1000 colony‐forming units per mL for urine cultures obtained by suprapubic aspiration, (2) 50,000 colony‐forming units per mL from a catheterized specimen, or (3) 10,000 colony‐forming units per mL from a catheterized specimen in association with a positive urinalysis.1214
Statistical Analysis
Data analysis was performed using STATA version 12 (Stata Corp, College Station, TX). Linear regression was used to determine the association between CSF RBC and CSF protein. We analyzed the following groups of children: 1) all eligible patients; 2) children 28 days versus children >28 days; 3) vaginal versus cesarean delivery; and 4) patients without CSF pleocytosis. In the primary subanalysis, CSF pleocytosis was defined as CSF white blood cells (WBCs) >19 cells/mm3 for infants 28 days of age and CSF WBCs >9 cells/mm3 for infants 29 days of age, using reference values established by Kestenbaum et al.10 Alternate definitions of CSF pleocytosis were also examined using reference values proposed by Byington et al15 (age 28 days, >18 cells/mm3; age >29 days, >8.5 cells/mm3) and Chadwick et al16(age 0‐7 days, >26 cells/mm3; age 8‐28 days, >9 cells/mm3; age 29‐49 days, >8 cells/mm3; and age 50‐56 days, >7 cells/mm3). We did not correct CSF WBCs for the RBC count because prior studies suggest that such correction factors do not provide any advantage over uncorrected values.17 Finally, linear regression analysis was repeated while including subjects with >150,000 RBC/mm3 to determine the effect of including those patients on the association of CSF RBC count and protein concentrations. Subjects with grossly bloody CSF specimens, defined a priori as a CSF RBC >1,000,000/mm3, were excluded from this subanalysis.
RESULTS
There were 1986 infants, 56 days of age or younger, who underwent LP in the ED during the study period. Patients were excluded for the following reasons: missing medical record number (n = 16); missing CSF WBC, CSF RBC, or CSF protein values (n = 290); conditions known to elevate CSF protein concentrations (n = 426, as follows: presence of a ventricular shunt device [n = 48], serious bacterial infection [n = 149], congenital infection [n = 2], positive CSF polymerase chain reaction [PCR] test for either enterovirus or herpes simplex virus [n = 97], seizure prior to presentation [n = 98], or elevated serum bilirubin [n = 32]). An additional 13 patients with a CSF RBC count >150,000 cells/mm3 were also excluded.
For the remaining 1241 study infants, the median age was 34 days (interquartile range: 19 days‐46 days) and 554 patients (45%) were male. The median CSF RBC count was 40 cells/mm3 (interquartile range: 2‐1080 cells/mm3); 11.8% of patients had a CSF RBC count >10,000 cells/mm3.
CSF protein increased linearly with increasing CSF RBCs (Figure 1). The increase in the CSF protein concentration of 1.9 mg/dL per 1000 CSF RBCs for all patients was similar between different age groups and delivery types (Table 1). Restricting analysis to those patients without pleocytosis also yielded comparable results; applying 2 other definitions of pleocytosis did not change the magnitude of the association (Table 1).
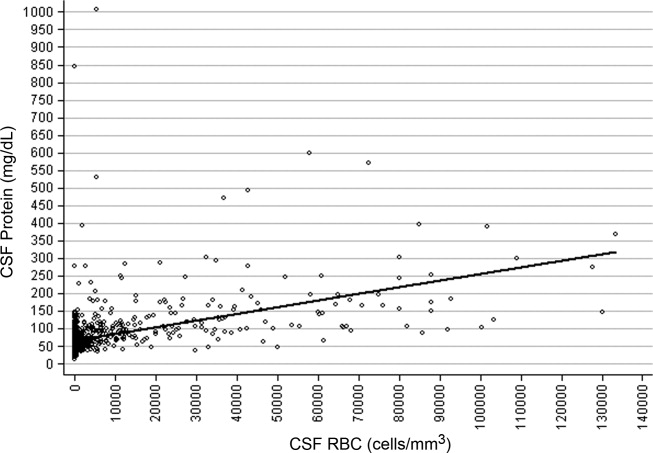
Patient Group | No. of Patients | Change in CSF protein (mg/dL) per 1000 RBCs (95% CI) |
---|---|---|
| ||
All eligible | 1241 | 1.9 (1.7‐2.1) |
No CSF pleocytosis* | 1085 | 2.0 (1.7‐2.4) |
Age | ||
Age 28 days | 481 | 1.9 (1.5‐2.3) |
Age >28 days | 760 | 1.9 (1.7‐2.1) |
Mode of delivery | ||
Vaginal | 741 | 1.9 (1.7‐2.2) |
Cesarean | 366 | 1.7 (1.4‐2.0) |
In a subanalysis, we then included subjects with a CSF RBC count >150,000/mm3; one extreme outlier with a CSF RBC equal to 3,160,000/mm3 remained excluded. Inclusion of more traumatic samples lessened the overall correction factor. The CSF protein increased by 1.22 mg/dL (95% confidence interval: 1.14‐1.29 mg/dL) per 1000 RBC/mm3 increase in the CSF. In the subset without CSF pleocytosis, the CSF protein increased by 1.44 mg/dL (95% confidence interval: 1.33‐1.57 mg/dL) per 1000 RBC/mm3.
Three children had high CSF protein values (>500 mg/dL) despite the relative paucity of CSF RBCs. Two of these infants had respiratory syncytial virus bronchiolitis; neither infant had signs or symptoms of neurological illness. While details of the labor and delivery were not available, the CSF sample for one of these infants was reported to have xanthochromia, and the other infant was reported to have had a traumatic LP with a CSF sample that subsequently cleared. The third infant had fever without a specific source identified, but had a birth history of vaginal delivery and prolonged labor. The CSF sample from LP for this patient was reported as grossly bloody by the performing clinicians and by the Clinical Microbiology Laboratory, despite a CSF red blood cell count of only 5500 cells/mm3.
DISCUSSION
In a large cohort of infants 56 days of age, CSF protein increased by approximately 2 mg/dL for every 1000 cell/mm3 increase in CSF RBCs. This correction factor is higher than previously reported correction factors from studies including older infants and children.6, 18 Some of this difference may be explained by the presence of old blood related to the trauma of labor and delivery. Previous work has demonstrated that the presence of xanthochromia, another RBC breakdown product, in the CSF of young infants was associated with maternal labor and elevated CSF protein.19 Consistent with this hypothesis, the correction factor was nominally higher in those infants born by vaginal delivery compared with those born by cesarean section.
Several infants in our study had high CSF protein levels despite a paucity of CSF RBCs. By convention at our institution, the protein and glucose values are determined from the second tube, and the WBCs and RBCs are determined from the third tube. However, we could not determine the order in which the specimens for protein and RBCs were collected for individual specimens. Additionally, it is possible that delayed clearance of blood from a traumatic LP would cause the CSF protein level to be high, as measured in the second tube, but lead to few RBCs in the third tube. These circumstances could explain the discrepancy between CSF protein and CSF RBCs counts for some patients.
The CSF protein adjustment factor for infants 56 days of age in our study was almost twice the correction of 1.1 mg/dL for every 1000 RBC increase reported by Nigrovic et al among infants 90 days of age.6 There are differences in the design of the 2 studies. We excluded subjects with exceedingly large numbers of CSF RBCs and restricted inclusion to those 56 days of age or younger. When subjects with >150,000 RBCs/mm3 were included, the correction decreased to a value comparable to that reported by Nigrovic et al.6 Therefore, it is possible that inclusion of subjects with grossly bloody specimens in prior studies skewed the association between CSF protein and CSF RBCs. The number of subjects in our cohort with >150,000 CSF RBCs was too small to calculate a relevant correction factor for infants with exceedingly high CSF RBC counts.
The results of this study should be considered in the context of several limitations. Details regarding labor and delivery were not available. We suspect that old blood related to the trauma of birth provides partial explanation for the higher correction factor in neonates and young infants compared with older children. However, differences in CSF blood‐brain barrier permeability may also contribute to these differences, independent of the CSF RBC count. Additionally, though the study population included a large number of neonates and young infants, a relatively small proportion of subjects had high CSF RBC counts. Therefore, our results may not be generalizable to those with exceedingly high CSF RBCs. Finally, available clinical prediction rules to identify patients with CSF pleocytosis, who are at very low risk for bacterial meningitis, include CSF protein as a predictor.3, 20, 21 Although CSF protein in children with traumatic LPs may need adjustment prior to application of the clinical prediction rule, further study is needed before implementing this approach.
In conclusion, we found that CSF protein concentrations increased by approximately 2 mg/dL for every 1000 CSF RBCs. Correction of CSF protein for those with extremely high CSF RBCs may not be appropriate, as conventional linear models do not apply. These data may assist clinicians in interpreting CSF protein concentrations in infants 56 days of age and younger in the context of traumatic LPs.
Traumatic lumbar puncture (LP) occurs when peripheral blood is introduced into the cerebrospinal fluid (CSF) as a result of needle trauma, which causes bleeding into the subarachnoid space. Traumatic LPs occur in up to 30% of LPs performed in children.1, 2 In addition to affecting the CSF white blood cell count, the presence of CSF red blood cells (RBCs) is associated with higher CSF protein concentrations due to the higher protein concentration in plasma compared with CSF and to the release of protein from lysed red blood cells. CSF protein concentration has been used in clinical decision rules for the prediction of bacterial meningitis in children.3 Elevated protein levels are difficult to interpret in cases of traumatic LP, and a diagnosis of bacterial meningitis may be more difficult to exclude on the basis of CSF test results.4
The interpretation of CSF protein levels is further complicated in the youngest infants due to both the changing composition of the CSF as well as the higher rates of traumatic LPs.5 Therefore, studies establishing a correction factor, adjusting observed CSF protein levels for the presence of CSF RBCs, that included predominantly older children may not be generalizable to neonates and young infants.6 We sought to determine the relationship between CSF RBC count and CSF protein in infants 56 days of age who underwent LP in the emergency department (ED).
METHODS
Study Design, Setting, and Participants
This cross‐sectional study was performed at The Children's Hospital of Philadelphia (Philadelphia, PA), an urban, tertiary care children's hospital. The Committees for the Protection of Human Subjects approved this study with a waiver of informed consent.
Infants 56 days of age and younger were eligible for inclusion if they had an LP performed as part of their ED evaluation between January 1, 2005 and July 31, 2009. At The Children's Hospital of Philadelphia, infants 56 days and younger routinely receive LPs for evaluation of fever.79 Patients undergoing LP in the ED were identified using computerized order entry records as previously described.5, 10
We excluded patients with conditions known to elevate CSF protein, including: serious bacterial infection (bacterial meningitis, urinary tract infection, bacteremia, pneumonia, septic arthritis, and bacterial gastroenteritis),11 presence of a ventricular shunt, aseptic meningitis (positive CSF enteroviral polymerase chain reaction or CSF herpes simplex virus polymerase chain reaction), congenital infections (eg, syphilis), seizure prior to presentation, and elevated bilirubin (if serum bilirubin was obtained). Due to the fact that grossly bloody CSF samples are difficult to interpret, we excluded those with a CSF RBC count >150,000 cells/mm3, a cutoff representing the 99th percentile of CSF RBC values in the cohort after applying other exclusion criteria.
Study Definitions
Bacterial meningitis was defined as either the isolation of a known bacterial pathogen from the CSF or, in patients who received antibiotics prior to evaluation, the combination of CSF pleocytosis and bacteria reported on CSF Gram stain. Bacteremia was defined as the isolation of a known bacterial pathogen from blood cultures excluding commensal skin flora. Urinary tract infection was defined as growth of a single known pathogen meeting 1 of 3 criteria: (1) 1000 colony‐forming units per mL for urine cultures obtained by suprapubic aspiration, (2) 50,000 colony‐forming units per mL from a catheterized specimen, or (3) 10,000 colony‐forming units per mL from a catheterized specimen in association with a positive urinalysis.1214
Statistical Analysis
Data analysis was performed using STATA version 12 (Stata Corp, College Station, TX). Linear regression was used to determine the association between CSF RBC and CSF protein. We analyzed the following groups of children: 1) all eligible patients; 2) children 28 days versus children >28 days; 3) vaginal versus cesarean delivery; and 4) patients without CSF pleocytosis. In the primary subanalysis, CSF pleocytosis was defined as CSF white blood cells (WBCs) >19 cells/mm3 for infants 28 days of age and CSF WBCs >9 cells/mm3 for infants 29 days of age, using reference values established by Kestenbaum et al.10 Alternate definitions of CSF pleocytosis were also examined using reference values proposed by Byington et al15 (age 28 days, >18 cells/mm3; age >29 days, >8.5 cells/mm3) and Chadwick et al16(age 0‐7 days, >26 cells/mm3; age 8‐28 days, >9 cells/mm3; age 29‐49 days, >8 cells/mm3; and age 50‐56 days, >7 cells/mm3). We did not correct CSF WBCs for the RBC count because prior studies suggest that such correction factors do not provide any advantage over uncorrected values.17 Finally, linear regression analysis was repeated while including subjects with >150,000 RBC/mm3 to determine the effect of including those patients on the association of CSF RBC count and protein concentrations. Subjects with grossly bloody CSF specimens, defined a priori as a CSF RBC >1,000,000/mm3, were excluded from this subanalysis.
RESULTS
There were 1986 infants, 56 days of age or younger, who underwent LP in the ED during the study period. Patients were excluded for the following reasons: missing medical record number (n = 16); missing CSF WBC, CSF RBC, or CSF protein values (n = 290); conditions known to elevate CSF protein concentrations (n = 426, as follows: presence of a ventricular shunt device [n = 48], serious bacterial infection [n = 149], congenital infection [n = 2], positive CSF polymerase chain reaction [PCR] test for either enterovirus or herpes simplex virus [n = 97], seizure prior to presentation [n = 98], or elevated serum bilirubin [n = 32]). An additional 13 patients with a CSF RBC count >150,000 cells/mm3 were also excluded.
For the remaining 1241 study infants, the median age was 34 days (interquartile range: 19 days‐46 days) and 554 patients (45%) were male. The median CSF RBC count was 40 cells/mm3 (interquartile range: 2‐1080 cells/mm3); 11.8% of patients had a CSF RBC count >10,000 cells/mm3.
CSF protein increased linearly with increasing CSF RBCs (Figure 1). The increase in the CSF protein concentration of 1.9 mg/dL per 1000 CSF RBCs for all patients was similar between different age groups and delivery types (Table 1). Restricting analysis to those patients without pleocytosis also yielded comparable results; applying 2 other definitions of pleocytosis did not change the magnitude of the association (Table 1).
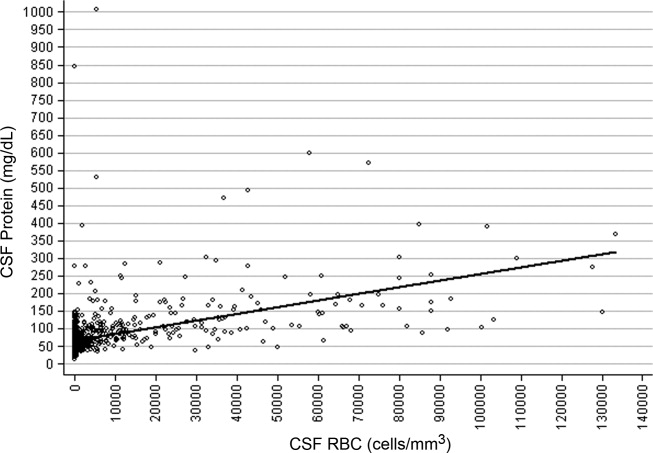
Patient Group | No. of Patients | Change in CSF protein (mg/dL) per 1000 RBCs (95% CI) |
---|---|---|
| ||
All eligible | 1241 | 1.9 (1.7‐2.1) |
No CSF pleocytosis* | 1085 | 2.0 (1.7‐2.4) |
Age | ||
Age 28 days | 481 | 1.9 (1.5‐2.3) |
Age >28 days | 760 | 1.9 (1.7‐2.1) |
Mode of delivery | ||
Vaginal | 741 | 1.9 (1.7‐2.2) |
Cesarean | 366 | 1.7 (1.4‐2.0) |
In a subanalysis, we then included subjects with a CSF RBC count >150,000/mm3; one extreme outlier with a CSF RBC equal to 3,160,000/mm3 remained excluded. Inclusion of more traumatic samples lessened the overall correction factor. The CSF protein increased by 1.22 mg/dL (95% confidence interval: 1.14‐1.29 mg/dL) per 1000 RBC/mm3 increase in the CSF. In the subset without CSF pleocytosis, the CSF protein increased by 1.44 mg/dL (95% confidence interval: 1.33‐1.57 mg/dL) per 1000 RBC/mm3.
Three children had high CSF protein values (>500 mg/dL) despite the relative paucity of CSF RBCs. Two of these infants had respiratory syncytial virus bronchiolitis; neither infant had signs or symptoms of neurological illness. While details of the labor and delivery were not available, the CSF sample for one of these infants was reported to have xanthochromia, and the other infant was reported to have had a traumatic LP with a CSF sample that subsequently cleared. The third infant had fever without a specific source identified, but had a birth history of vaginal delivery and prolonged labor. The CSF sample from LP for this patient was reported as grossly bloody by the performing clinicians and by the Clinical Microbiology Laboratory, despite a CSF red blood cell count of only 5500 cells/mm3.
DISCUSSION
In a large cohort of infants 56 days of age, CSF protein increased by approximately 2 mg/dL for every 1000 cell/mm3 increase in CSF RBCs. This correction factor is higher than previously reported correction factors from studies including older infants and children.6, 18 Some of this difference may be explained by the presence of old blood related to the trauma of labor and delivery. Previous work has demonstrated that the presence of xanthochromia, another RBC breakdown product, in the CSF of young infants was associated with maternal labor and elevated CSF protein.19 Consistent with this hypothesis, the correction factor was nominally higher in those infants born by vaginal delivery compared with those born by cesarean section.
Several infants in our study had high CSF protein levels despite a paucity of CSF RBCs. By convention at our institution, the protein and glucose values are determined from the second tube, and the WBCs and RBCs are determined from the third tube. However, we could not determine the order in which the specimens for protein and RBCs were collected for individual specimens. Additionally, it is possible that delayed clearance of blood from a traumatic LP would cause the CSF protein level to be high, as measured in the second tube, but lead to few RBCs in the third tube. These circumstances could explain the discrepancy between CSF protein and CSF RBCs counts for some patients.
The CSF protein adjustment factor for infants 56 days of age in our study was almost twice the correction of 1.1 mg/dL for every 1000 RBC increase reported by Nigrovic et al among infants 90 days of age.6 There are differences in the design of the 2 studies. We excluded subjects with exceedingly large numbers of CSF RBCs and restricted inclusion to those 56 days of age or younger. When subjects with >150,000 RBCs/mm3 were included, the correction decreased to a value comparable to that reported by Nigrovic et al.6 Therefore, it is possible that inclusion of subjects with grossly bloody specimens in prior studies skewed the association between CSF protein and CSF RBCs. The number of subjects in our cohort with >150,000 CSF RBCs was too small to calculate a relevant correction factor for infants with exceedingly high CSF RBC counts.
The results of this study should be considered in the context of several limitations. Details regarding labor and delivery were not available. We suspect that old blood related to the trauma of birth provides partial explanation for the higher correction factor in neonates and young infants compared with older children. However, differences in CSF blood‐brain barrier permeability may also contribute to these differences, independent of the CSF RBC count. Additionally, though the study population included a large number of neonates and young infants, a relatively small proportion of subjects had high CSF RBC counts. Therefore, our results may not be generalizable to those with exceedingly high CSF RBCs. Finally, available clinical prediction rules to identify patients with CSF pleocytosis, who are at very low risk for bacterial meningitis, include CSF protein as a predictor.3, 20, 21 Although CSF protein in children with traumatic LPs may need adjustment prior to application of the clinical prediction rule, further study is needed before implementing this approach.
In conclusion, we found that CSF protein concentrations increased by approximately 2 mg/dL for every 1000 CSF RBCs. Correction of CSF protein for those with extremely high CSF RBCs may not be appropriate, as conventional linear models do not apply. These data may assist clinicians in interpreting CSF protein concentrations in infants 56 days of age and younger in the context of traumatic LPs.
- Local anesthetic and stylet styles: factors associated with resident lumbar puncture success.Pediatrics.2006;117:876–881. , , , , , .
- Risk factors for traumatic or unsuccessful lumbar punctures in children.Ann Emerg Med.2007;49:762–771. , , .
- Clinical prediction rule for identifying children with cerebrospinal fluid pleocytosis at very low risk of bacterial meningitis.JAMA.2007;297:52–60. , , , et al.
- Interpretation of traumatic lumbar punctures: who can go home?Pediatrics.2003;111:525–528. , , .
- Age‐specific reference values for cerebrospinal fluid protein concentration in neonates and young infants.J Hosp Med.2011;6:22–27. , , , , .
- Correction of cerebrospinal fluid protein for the presence of red blood cells in children with a traumatic lumbar puncture.J Pediatr.2011;159:158–159. , , .
- Failure of infant observation scales in detecting serious illness in febrile, 4‐ to 8‐week‐old infants.Pediatrics.1990;85:1040–1043. , , .
- Unpredictability of serious bacterial illness in febrile infants from birth to 1 month of age.Arch Pediatr Adolesc Med.1999;153:508–511. , .
- Outpatient management without antibiotics of fever in selected infants.N Engl J Med.1993;329:1437–1441. , , .
- Defining cerebrospinal fluid white blood cell count reference values in neonates and young infants.Pediatrics.2010;125:257–264. , , , , .
- Sterile cerebrospinal fluid pleocytosis in young infants with urinary tract infections.J Pediatr.2008;153:290–292. , , , , .
- Clinical and demographic factors associated with urinary tract infection in young febrile infants.Pediatrics.2005;116:644–648. , , , et al.
- Prevalence of urinary tract infection in febrile young children in the emergency department.Pediatrics.1998;102:e16. , , , , .
- Prevalence of urinary tract infection in febrile infants.J Pediatr.1993;123:17–23. , , , , , .
- Normative cerebrospinal fluid profiles in febrile infants.J Pediatr.2011;158:130–134. , , .
- Cerebrospinal fluid characteristics of infants who present to the emergency department with fever: establishing normal values by week of age.Pediatr Infect Dis J.2011;30:e63–e67. , , , .
- Corrections for leukocytes and percent of neutrophils do not match observations in blood‐contaminated cerebrospinal fluid and have no value over uncorrected cells for diagnosis.Pediatr Infect Dis J.2006;25:8–11. , .
- Distinguishing cerebrospinal fluid abnormalities in children with bacterial meningitis and traumatic lumbar puncture.J Infect Dis.1990;162:251–254. , , , , .
- Cerebrospinal fluid xanthochromia in newborns is related to maternal labor before delivery.Pediatrics.2007;120:e1212–e1216. , , , .
- Accuracy and test characteristics of ancillary tests of cerebrospinal fluid for predicting acute bacterial meningitis in children with low white blood cell counts in cerebrospinal fluid.Acad Emerg Med.2005;12:303–309. , .
- A decision rule for predicting bacterial meningitis in children with cerebrospinal fluid pleocytosis when gram stain is negative or unavailable.Acad Emerg Med.2008;15:437–444. , , , .
- Local anesthetic and stylet styles: factors associated with resident lumbar puncture success.Pediatrics.2006;117:876–881. , , , , , .
- Risk factors for traumatic or unsuccessful lumbar punctures in children.Ann Emerg Med.2007;49:762–771. , , .
- Clinical prediction rule for identifying children with cerebrospinal fluid pleocytosis at very low risk of bacterial meningitis.JAMA.2007;297:52–60. , , , et al.
- Interpretation of traumatic lumbar punctures: who can go home?Pediatrics.2003;111:525–528. , , .
- Age‐specific reference values for cerebrospinal fluid protein concentration in neonates and young infants.J Hosp Med.2011;6:22–27. , , , , .
- Correction of cerebrospinal fluid protein for the presence of red blood cells in children with a traumatic lumbar puncture.J Pediatr.2011;159:158–159. , , .
- Failure of infant observation scales in detecting serious illness in febrile, 4‐ to 8‐week‐old infants.Pediatrics.1990;85:1040–1043. , , .
- Unpredictability of serious bacterial illness in febrile infants from birth to 1 month of age.Arch Pediatr Adolesc Med.1999;153:508–511. , .
- Outpatient management without antibiotics of fever in selected infants.N Engl J Med.1993;329:1437–1441. , , .
- Defining cerebrospinal fluid white blood cell count reference values in neonates and young infants.Pediatrics.2010;125:257–264. , , , , .
- Sterile cerebrospinal fluid pleocytosis in young infants with urinary tract infections.J Pediatr.2008;153:290–292. , , , , .
- Clinical and demographic factors associated with urinary tract infection in young febrile infants.Pediatrics.2005;116:644–648. , , , et al.
- Prevalence of urinary tract infection in febrile young children in the emergency department.Pediatrics.1998;102:e16. , , , , .
- Prevalence of urinary tract infection in febrile infants.J Pediatr.1993;123:17–23. , , , , , .
- Normative cerebrospinal fluid profiles in febrile infants.J Pediatr.2011;158:130–134. , , .
- Cerebrospinal fluid characteristics of infants who present to the emergency department with fever: establishing normal values by week of age.Pediatr Infect Dis J.2011;30:e63–e67. , , , .
- Corrections for leukocytes and percent of neutrophils do not match observations in blood‐contaminated cerebrospinal fluid and have no value over uncorrected cells for diagnosis.Pediatr Infect Dis J.2006;25:8–11. , .
- Distinguishing cerebrospinal fluid abnormalities in children with bacterial meningitis and traumatic lumbar puncture.J Infect Dis.1990;162:251–254. , , , , .
- Cerebrospinal fluid xanthochromia in newborns is related to maternal labor before delivery.Pediatrics.2007;120:e1212–e1216. , , , .
- Accuracy and test characteristics of ancillary tests of cerebrospinal fluid for predicting acute bacterial meningitis in children with low white blood cell counts in cerebrospinal fluid.Acad Emerg Med.2005;12:303–309. , .
- A decision rule for predicting bacterial meningitis in children with cerebrospinal fluid pleocytosis when gram stain is negative or unavailable.Acad Emerg Med.2008;15:437–444. , , , .
Copyright © 2012 Society of Hospital Medicine