User login
The Burden of Cardiac Complications in Patients with Community-Acquired Pneumonia
From the Division of Infectious Diseases, School of Medicine, University of Louisville, Louisville, KY.
Abstract
- Objective: To summarize the published literature on cardiac complications in patients with community-acquired pneumonia (CAP) as well as provide a historical context for the topic; and to provide recommendations concerning preventing and anticipating cardiac complications in patients with CAP.
- Methods: Literature review.
- Results: CAP patients are at increased risk for arrhythmias (~5%), myocardial infarction (~5%), and congestive heart failure (~14%). Oxygenation, the level of heart conditioning, local (pulmonary) and systemic (cytokines) inflammation, and medication all contribute to the pathophysiology of cardiac complications in CAP patients. A high Pneumonia Severity Index (PSI) can be used to screen for risk of cardiac complications in CAP patients; however, a new but less studied clinical rule developed to risk stratify patient hospitalized for CAP was shown to outperform the PSI. A troponin test and ECG should be obtained in all patients admitted for CAP while a cardiac echocardiogram may be reserved for higher-risk patients.
- Conclusions: Cardiac complications, including arrhythmias, myocardial infarctions, and congestive heart failure, are a significant burden among patients hospitalized for CAP. Influenza and pneumococcal vaccination should be emphasized among appropriate patients. Preliminary data suggest that those with CAP may be helped if they are already on aspirin or a statin. Early recognition of cardiac complications and treatment may improve clinical outcomes for patients with CAP.
Community-acquired pneumonia (CAP) is a common condition in the United States and a leading cause of morbidity and mortality [1,2], with medical costs exceeding $10 billion in 2011 [3]. The mortality rate is much higher for those aged 65 years and older [4]. Men have a higher death rate than women (18.6 vs. 13.9 per 100,000 population), and death rate varies based on ethnicity, with mortality rates for American Indian/Alaska natives at 19.2, blacks at 17.1, whites at 15.9, Asian/Pacific Islanders at 15.0, and Hispanics at 13.1 (all rates per 100,000) [2]. CAP causes considerable worldwide mortality, with differences in mortality varying according to world region [5].
Cardiovascular complications and death from other comorbidities cause a substantial proportion of CAP-associated mortality. In Mortensen et al’s study, among patients with CAP who died, at least one third had a cardiac complication, and 13% had a cardiac-related cause of death [6]. One study showed that hospitalized patients with CAP complicated by heart disease were 30% more likely to die than patients hospitalized with CAP alone [7]. In this article, we discuss the burden of cardiac complications in adults with CAP, including underlying pathophysiological processes and strategies to prevent their occurence.
Pathophysiological Processes of Heart Disease Caused by CAP
The pathophysiology of cardiac complications as a result of CAP is made up of several hypotheses, including (1) declining oxygen provision by the lungs in the face of increasing demand by the heart, (2) a lack of reserve for stress because of cardiac comorbidities and (3) localized (pulmonary) inflammation leading to systemic (including cardiac) complications by the release of cytokines or other chemicals. Any of these may result in cardiac complications occurring before, during, or after a patient has been hospitalized for CAP. Antimicrobial treatment, specifically azithromycin, has also been implicated in myocardial adverse effects. Although azithromycin is most noted for causing QT prolongation, it was associated with myocardial infarction (MI) in a study of 73,690 patients with pneumonia [8]. A higher proportion of those who received azithromycin had an MI compared to those who did not (5.1% vs 4.4%; OR 1.17; 95% CI, 1.08–1.25), but there was no statistical difference in cardiac arrhythmias, and the 90-day mortality was actually better in the azithromycin group (17.4% vs 22.3%; odds ratio [OR], 0.73; 95% CI, 0.70–0.76).
Systemic inflammation is the result of several molecules, such as cytokines, chemokines and reactive oxidant species. Reactive oxidant species may determine oxidation of proteins, lipids and DNA, which leads to cell death. The hypothesis also purports that they also cause destabilization of atherosclerotic plaques leading to MIs. Other reactions as a result of inflammation lead to arrhythmias with or without compromised cardiac function, causing congestive heart failure (CHF). For this reason, some authors have approached the pathophysiology of cardiac complications by considering them to be either plaque-related or plaque-unrelated events [9].
A few studies have linked specific inflammatory molecules to cardiac toxicity. NOX2 is chemically unstable and may provoke cellular damage, thus maintaining a certain redox balance is crucial for cardiomyocyte health. In 248 patients with CAP, an elevated troponin T was present in 135 patients and among those, NOX2 correlated with the troponin T values (OR 1.13, 95% CI 1.08–1.17; P < 0.001) [10]. Both disrupting the equilibrium of the redox balance by upregulating NOX2, and finding NOX2 to be associated with troponin T suggest that oxidative stress is implicated in damage to the myocardium during CAP. In another study of 432 patients with CAP, 41 developed atrial fibrillation within 24 to 72 hours of admission and showed higher blood levels of NOX2 than those who had CAP without atrial fibrillation [11]. Oxidative stress has been shown to cause hypertrophy, dysfunction, apoptotic cell death, and fibrosis in the myocardium [12].
There is likely a high level of variability in how individual patients respond to a predisposing factor for a cardiac complication. For example, one patient may tolerate a mild hypoxia while another is sensitive. The association of inflammatory markers with the presence of cardiac markers, however, would support that once there are systemic reactions, the complications increase. Macrolides, however, were not found to contribute to long-term mortality due to cardiac complications.
Cardiac Complications of CAP
After the H1N1 influenza outbreak of 1918, it was noted that all-cause mortality increased during the outbreak as did influenza-related deaths. This prompted inquiry as to whether there was an actual association between the outbreak and increased overall mortality, or whether the 2 occurrences were simply coincidental [14]. Near that time, arrhythmias in CAP patients were studied. T-wave changes were found to be associated with CAP [15]. Among 92 patients studied, 449 electrocardiograms (ECGs) were reviewed. T-wave changes were the most common ECG changes. They were found in 5 of 10 of the patients who died, and in 35 of the 82 patients who lived. Twelve living patients had persistent ECG changes, and although they were all thought to have had underlying myocardial disease, 2 of them certainly did as they each had an acute MI (and the ECG was included as a figure for one of them).
A study in the 1980s that reported 3 of 38 CAP patients with CHF interrupted the paucity of data at the time that showed that having a cardiac complication during CAP was a known entity [16]. By the end of the 20th century, Meier et al noted that among case patients who had an MI, an acute respiratory tract infection preceded the MI in 2.8% while in only 0.9% of control patients [17]. They also noted that patients who had an acute respiratory tract infection were 2.7 times more likely to have an MI in the following 10 days than control patients.
Further study by Musher et al revealed that MI was associated with pneumococcal pneumonia in 12 (7%) of 170 veteran patients [18]. An MI was defined on the basis of ECG abnormalities (Q waves or ST segment elevation or depression) with troponin I levels ≥ 0.5 ng/mL. They also evaluated arrhythmias and CHF. They included atrial fibrillation or flutter and ventricular tachycardia while excluding terminal arrhythmias. An arrhythmia was found in 8 (5%) patients. CHF was based on Framingham criteria (Table 1) [19]. New or worsening CHF was determined by comparing physical findings, laboratory values, chest radiograph, and echocardiogram reports in medical records. CHF was found in 13 (19%) patients. Ramirez et al found that MI was associated with CAP in 29 (5.8%) of 500 similar veteran patients [20].
Corrales-Medina et al reported cardiac complications in CAP patients in the Pneumonia Patient Outcomes Team cohort study [21]. They defined MI as the presence of 2 of 3 criteria: ECG abnormalities, elevated cardiac enzymes, and chest pain. They found 43 (3.2%) of 1343 patients with an MI. Arrhythmias included atrial fibrillation or flutter, multifocal atrial tachycardia, supraventricular tachycardia, ventricular tachycardia (≥ 3 beat run) or ventricular fibrillation. With the more inclusive list, they found a greater proportion, 137 (10%) patients affected. They defined CHF with physical examination findings plus a radiographic abnormality, and found 279 (21%) patients affected. A meta-analysis of 17 studies had pooled incidences for an MI of 5.3%, an arrhythmia of 4.7% and CHF of 14.1% [22].
In summary, the most prominent cardiac complications in patients with CAP have been found to be CHF, MI, and arrhythmia.
Timing of Cardiac Complications in Relation to CAP
While a patient is still in the community, cardiac complications may occur with the onset of CAP, or afterwards. For these patients, the primary goal is to identify the complication and manage it as soon as the patient is admitted for CAP, rather than allowing the complications to worsen only to be recognized later. Cardiac complications are rare in outpatients overall. A study of 944 outpatients found heart failure in 1.4%, arrhythmias in 1.0% and MI in 0.1% [21].
For patients who are admitted with CAP but who do not have a cardiac complication, the goals are either to prevent any complication or to recognize and manage a complication early. This also applies to patients who have been discharged after an admission for CAP. Cardiac complications have been recorded shortly after (within 30 days), and late (up to 1 year) after discharge. A study of over 50,000 veterans who were admitted for CAP were followed for any cardiovascular complication in the next 90 days. Approximately 7500 veterans were found to have a cardiac complication, including (in order of highest to lowest frequency) CHF, arrhythmia, MI, stroke and angina [23]. More than 75% of the complications were found on the day of hospitalization, but events were still measured at 30 days and 90 days.
Two other studies sought to determine an association between CAP and cardiac complications differently; not by following CAP patients prospectively for complications but by retrospectively evaluating patients for a respiratory infection among those who were admitted for a cardiovascular complication (MI or stroke). A study of over 35,000 first-time admissions for either an MI or a stroke were evaluated for a respiratory infection within the previous 90 days [24]. The incidence rates were statistically significant for every time period up to 90 days. The preceding 3 days was the time period with the highest frequency for a respiratory infection preceding an event. When the event was an MI, the incident rate was 4.95 (95% CI, 4.43–5.53). A similar study of over 20,000 first-time admissions for either an MI or stroke were evaluated for a preceding primary care visit for a respiratory infection [25]. An infection preceded 2.9% of patients with an MI and 2.8% of patients with a stroke. Statistical significance was found for the group of patients who had a respiratory infection within 7 days preceding an MI (OR 2.10 [95% CI 1.38–3.21]) or preceding a stroke (OR 1.92 [95% CI 1.24–2.97]). In fact, every time period analyzed for both complications (MI and stroke) was significant up to 1 year. Because the timing of a cardiac complication varies and can occur up to 90 days or even a year after acute infection, physicians should maintain vigilance in suspecting and screening for them.
Predictors of Cardiac Complications During CAP
Recently, Cangemi et al reviewed mortality in 301 patients admitted for CAP 6 to 60 months after they were discharged [26]. Mortality was compared between patients who experienced a cardiac complication—atrial fibrillation or an ST- or non-ST-elevation MI—during their admission and those who did not. A total of 55 (18%) patients had a cardiac complication while hospitalized. During the follow-up, 90 (30%) of the 301 patients died. Death occurred in more patients who had had a cardiac complication while hospitalized than in those who did not (32% vs 13%; P < 0.001). The study also showed that age and the pneumonia severity index (PSI) predicted death in addition to intra-hospital complication. A Cox regression analysis showed that intrahospital cardiac complications (hazard ratio [HR] 1.76 [95% CI 1.10–2.82]; P = 0.019), age (HR 1.05 [95% CI 1.03–1.08]; P < 0.001) and the PSI (HR 1.01 [95% CI 1.00–1.02] P = 0.012) independently predicted death after adjusting for possible confounders [26].
The PSI score was published in 1997, and it instructed that patients with a risk class of I or II (low risk) should be managed as outpatients. Data eventually showed that there is a portion of the population with a risk class of I or II whose hospital admission is justified [4]. Among the reasons found was “comorbidity,” including MI and other cardiac complications. The PSI prediction rule was found to be useful in novel ways, and being associated with a risk of MI in patients with CAP was one of them. The propensity-adjusted association between the PSI score and MI was significant (P < 0.05) in an observational study of the CAP Organization (CAPO) [20]. Knowing that a PSI of 80 is in the middle of risk class III (71–90), it was noted that below 80 the risk for MI was zero to 2.5%, while above 80 the risk rose from 2.5% to 12.5%. A later study using the same statistical method showed a correlation between the PSI score and cardiac complications (MI, arrhythmias and CHF) with a P value of < 0.01 [21]. Determining the probability for the combination of complications, rather than just an MI, yielded an unsurprisingly higher range of risk for the PSI below 80, which was zero to 17.5%, while risk for a PSI above 80 was 17.5% to 80%.
In a study to determine risk factors for cardiac complications among 3068 patients with CAP, Griffin et al applied a purposeful selection algorithm to a list of factors with reasonable potential to be associated with the 376 patients who actually had a cardiac complication [27]. After multivariate logistic regression analysis, hyperlipidemia, an infection with Staphlococcus aureus or Klebsiella pneumoniae, and the PSI were found to be statistically significant. In contrast, statin therapy was associated with a lower risk of an event.
In 2014, a validated score similar to the PSI and using the same database was derived to predict short-term risk for cardiac events in hospitalized patients with CAP [28]. It attributes points for age, 3 preexisting conditions, 2 vital signs and 7 radiological and laboratory values, with a point scoring system that defines 4 risk stratification classes. In the derivation cohort, the incidence of cardiac complications across the risk classes increased linearly (3%, 18%, 35%, and 72%, respectively). The score was validated in the original publication with a separate database but has not been evaluated since. The score outperformed the PSI score in predicting cardiac complications in the validation cohort (proportion of patients correctly reclassified by the new score, 44%). Potentially, the rule could help identify high-risk patients upon admission and could assist clinicians in their decision making.
Strategies to Prevent Cardiac Complications During CAP
It is now well established that there is a heavy burden of long-lasting cardiac complications among patients with CAP; therefore, preventing CAP should be a priority. This can be accomplished by counseling patients to refrain from alcohol and smoking and by administering influenza (Table 2) and pneumococcal vaccines (Figure 2). Since the 7-valent protein-polysaccharide conjugate pneumococcal vaccine (PCV-7) was released for children in 2000, there have been fewer hospitalizations in the United States [27] and improved outcomes globally;
for instance, fewer hospitalizations among children < 14 years of age in Uruguay [29], and decreased invasive pneumococcal disease among children < 5 years of age in Taiwan [30]. Furthermore, a decrease in invasive pneumococcal disease by 18% in persons aged > 65 years in the US and Canada decreased with the introduction of PCV-7 to children. Although this showed a beneficial indirect effect (herd immunity) in unvaccinated populations [31,32], there have been no randomized controlled trials in adults demonstrating a decrease in pneumococcal pneumonia or invasive pneumococcal disease which were vaccinated with PCV-13. The Food and Drug Administration approved PCV-13 for children in 2010 and for adults in 2012. Although it included fewer serotypes, it did include serotype 6A, which has a high pathogenicity and is not in 23-valent pneumococcal polysaccharide vaccine (PPSV-23). The criteria for vaccinating adults for pneumococcal infection were recently published [33]. A study of patients with invasive pneumococcal disease, which also determined pneumococcal serotypes, included 5 patients who had CAP as well [34]. Those patients had serotypes 6A, 7C, 14, and 23F (2 patients). The patient who had serotype 14 (higher pathogenicity) died and the other 4 lived. Serotypes 14 and 23F are in both vaccines while serotype 7C is in neither. Vaccination status was not provided in the study. At this time, there is evidence to support vaccinating patients for both S. pneumoniae and influenza virus.
Two methods used to prevent cardiac complications in general have been administration of aspirin and statins. The anticlotting properties of aspirin help to maintain blood flow in arteries narrowed by atherosclerosis. A meta-analysis of 10 randomized controlled trials found a statistically significant association between aspirin and a benefit on nonfatal myocardial infarctions/coronary events [35]. The associations were found with doses of 100 mg or less daily, and benefits were seen within 1 to 5 years. Statins have also been found to reduce all-cause mortality, cardiac-related mortality, and myocardial infarction [36]. A statin may stabilize coronary artery plaques that otherwise may rupture and cause myocardial ischemia or an infarct. But statins have also been found to be associated with a decreased risk of CAP. A comprehensive systematic review and meta-analysis found a decreased risk of CAP (OR 0.84; 95% CI, 0.74– 0.95) and decreased short-term mortality in patients with CAP (OR 0.68; 95% CI, 0.56–0.78) as a result of statin therapy [37]. The studies included any of 8 available statins. A prospective observational study found that patients who had been on a statin prior to being admitted for CAP had lower mortality, a lower incidence of complicated pneumonia and a lower C-reactive protein [38]. The lower C-reactive protein identifies decreased inflammation, which translates into improved endothelial function, modulated antioxidant effects, and a reduction in pro-inflammatory cytokines, hence its association with less severe CAP. Further study may reveal that a certain patient population should receive a statin to prevent CAP and improve outcomes. Overall, data support taking aspirin to prevent cardiac events regardless of CAP; further investigation of the benefits of statins to prevent cardiac complications in CAP patients is needed.
Clinical Applications
There are several implications of knowing the relationship between cardiac complications and CAP. First, physicians can better inform their patients about risks once they have been diagnosed with pneumonia. Second, physicians may be more likely to recognize a complication early and provide appropriate intervention. Third, physicians can risk stratify patients using the prediction score for cardiac complications in CAP patients [28]. In 1931 Master et al found that some patients with CAP also had PR interval or T-wave changes present for about 3 days, so they recommended obtaining an ECG to determine when a patient might be able to be discharged or declared “cured” [39]. Now, we are similarly recommending obtaining an ECG in CAP patients, but upon admission, in order to identify those who may get ischemic changes, arrhythmias or QTc prolongations. Pro-brain natriuretic peptide and troponins may be obtained independently of ECG results, and a cardiac echocardiogram may be reserved for those with a high risk of complications [40]. Finally, we recommend screening all patients for need for influenza and pneumococcal vaccines and administering according to the Advisory Committee on Immunization Practices of the Centers for Disease and Prevention [33].
Research Implications
The fact that cardiac complications in CAP patients is a well-defined entity with a significant degree of morbidity and mortality should prompt attentiona and resources to be directed to this area. The prediction score created specifically for this subpopulation of patients [28] can improve research by allowing adequate risk stratification to efficiently design and execute studies. Studies may be designed with fewer patients required to be enrolled while maintaining statistical power by limiting subject inclusion criteria to certain risk classes. Specific areas of future investigation should include the mechanisms of pathophysiology, which are not completely understood, and other complications, such as pulmonary edema, infectious endocarditis and pericarditis. Finally, cost has not been studied in this area or the potential savings of recognizing and preventing cardiac complications.
Summary
Cardiac complications, including arrhythmias, MI, and CHF are a significant burden among patients hospitalized for CAP. Influenza and pneumococcal vaccination should be emphasized among appropriate patients. The cardiac complication prediction score may be used to screen patients once admitted. A troponin and ECG should be obtained in all patients admitted for CAP while a cardiac echocardiogram may be reserved in higher-risk patients. Future research may be directed towards the subjects of pathophysiology other complications and cost.
Acknowledgment: We appreciate the critical review by Jessica Lynn Petrey, MSLS, Clinical Librarian, Kornhauser Health Sciences Library, University of Louisville, Louisville, KY.
Corresponding author: Dr. Forest Arnold, 501 E. Broadway, Suite 140 B, Louisville, KY 40202, f.arnold@louisville.edu
Financial disclosures: None.
1. Pfuntner A, Wier LM, Stocks C. HCUP statistical brief #162. Agency for Healthcare Research and Quality; Rockville, MD: 2013. Most frequent conditions in U.S. hospitals, 2011. Available at www.hcup-us.ahrq.gov/reports/statbriefs/sb162.pdf..
2. FastStats deaths and mortality. Centers for Disease Control and Prevention. Accessed 14 Oct 2015 at www.cdc.gov/nchs/fastats/deaths.htm.
3. Pfuntner A, Wier LM, Steiner C. HCUP statistical brief #168. Agency for Healthcare Research and Quality; Rockville, MD: 2013. Costs for hospital stays in the United States, 2011. Available at www.hcup-us.ahrq.gov/reports/statbriefs/sb168-Hospital-Costs-United-States-2011.pdf.
4. American Lung Association. Trends in pneumonia and influenza morbidity and mortality. November 2015. Available at
www.lung.org/assets/documents/research/pi-trend-report.pdf.
5. Arnold FW, Wiemken TL, Peyrani P, et al. Mortality differences among hospitalized patients with community-acquired pneumonia in three world regions: results from the Community-Acquired Pneumonia Organization (CAPO) International Cohort Study. Respir Med 2013;107:1101–11.
6. Mortensen EM, Coley CM, Singer DE, et al. Causes of death for patients with community-acquired pneumonia: results from the Pneumonia Patient Outcomes Research Team cohort study. Arch Intern Med 2002;162:1059–64.
7. Bordon J, Wiemken T, Peyrani P, et al. Decrease in long-term survival for hospitalized patients with community-acquired pneumonia. Chest 2010;138:279–83.
8. Mortensen EM, Halm EA, Pugh MJ, et al. Association of azithromycin with mortality and cardiovascular events among older patients hospitalized with pneumonia. JAMA 2014;311:2199–208.
9. Aliberti S, Ramirez JA. Cardiac diseases complicating community-acquired pneumonia. Curr Opin Infect Dis 2014;27:295–301.
10. Cangemi R, Calvieri C, Bucci T, et al. Is NOX2 upregulation implicated in myocardial injury in patients with pneumonia? Antioxid Redox Signal 2014;20:2949–54.
11. Violi F, Carnevale R, Calvieri C, et al. Nox2 up-regulation is associated with an enhanced risk of atrial fibrillation in patients with pneumonia. Thorax 2015;70:961–6.
12. Zhang Y, Tocchetti CG, Krieg T, Moens AL. Oxidative and nitrosative stress in the maintenance of myocardial function. Free Radic Biol Med 2012;53:1531–40.
13. Brown AO, Millett ER, Quint JK, Orihuela CJ. Cardiotoxicity during invasive pneumococcal disease. Am J Respir Crit Care Med 2015;191:739–45.
14. Collins SD. Excess mortality from causes other than influenza and pneumonia during influenza epidemics. Pub Health Rep 1932;47:2159–79.
15. Thomson KJ, Rustein DD, et al. Electrocardiographic studies during and after pneumococcus pneumonia. Am Heart J 1946;31:565–79.
16. Esposito AL. Community-acquired bacteremic pneumococcal pneumonia. Effect of age on manifestations and outcome. Arch Intern Med 1984;144:945–8.
17. Meier CR, Jick SS, Derby LE, et al. Acute respiratory-tract infections and risk of first-time acute myocardial infarction. Lancet 1998;351(9114):1467–71.
18. Musher DM, Rueda AM, Kaka AS, Mapara SM. The association between pneumococcal pneumonia and acute cardiac events. Clin Infect Dis 2007;45:158–65.
19. McKee PA, Castelli WP, McNamara PM, Kannel WB. The natural history of congestive heart failure: the Framingham study. N Engl J Med 1971;285:1441–6.
20. Ramirez J, Aliberti S, Mirsaeidi M, et al. Acute myocardial infarction in hospitalized patients with community-acquired pneumonia. Clin Infect Dis 2008;47:182–7.
21. Corrales-Medina VF, Musher DM, Wells GA, et al. Cardiac complications in patients with community-acquired pneumonia: incidence, timing, risk factors, and association with short-term mortality. Circulation 2012;125:773–81.
22. Corrales-Medina VF, Suh KN, Rose G, et al. Cardiac complications in patients with community-acquired pneumonia: a systematic review and meta-analysis of observational studies. PLoS Med 2011;8(6):e1001048.
23. Perry TW, Pugh MJ, Waterer GW, et al. Incidence of cardiovascular events after hospital admission for pneumonia. Am J Med 2011;124:244–51.
24. Smeeth L, Thomas SL, Hall AJ, et al. Risk of myocardial infarction and stroke after acute infection or vaccination. N Engl J Med 2004;351:2611–8.
25. Clayton TC, Thompson M, Meade TW. Recent respiratory infection and risk of cardiovascular disease: case-control study through a general practice database. Eur Heart J 2008;29:96–103.
26. Cangemi R, Calvieri C, Falcone M, et al. Relation of cardiac complications in the early phase of community-acquired pneumonia to long-term mortality and cardiovascular events. Am J Cardiol 2015;116:647–51.
27. Griffin MR, Zhu Y, Moore MR, et al. U.S. hospitalizations for pneumonia after a decade of pneumococcal vaccination. N Engl J Med 2013;369:155–63.
28. Corrales-Medina VF, Taljaard M, Fine MJ, et al. Risk stratification for cardiac complications in patients hospitalized for community-acquired pneumonia. Mayo Clin Proc 2014;89:60–8.
29. Pirez MC, Algorta G, Cedres A, et al. Impact of universal pneumococcal vaccination on hospitalizations for pneumonia and meningitis in children in Montevideo, Uruguay. Pediatr Infect Dis J 2011;30:669–74.
30. Liao WH, Lin SH, Lai CC, et al. Impact of pneumococcal vaccines on invasive pneumococcal disease in Taiwan. Eur J Clin Microbiol Infect Dis 2010;29:489–92.
31. Whitney CG, Farley MM, Hadler J, et al. Decline in invasive pneumococcal disease after the introduction of protein-polysaccharide conjugate vaccine. N Engl J Med 2003;348:1737–46.
32. Kellner JD, Church DL, MacDonald J, et al. Progress in the prevention of pneumococcal infection. CMAJ 2005;173:1149–51.
33. Kim DK, Bridges CB, Harriman KH, Advisory Committee on Immunization Practices. Recommended immunization schedule for adults aged 19 years or older: United States, 2016. Ann Intern Med 2016;164:184–94.
34. Kan B, Ries J, Normark BH, et al. Endocarditis and pericarditis complicating pneumococcal bacteraemia, with special reference to the adhesive abilities of pneumococci: results from a prospective study. Clin Microbiol Infect 2006;12:338–44.
35. Guirguis-Blake JM, Evans CV, Senger CA, et al. Aspirin for the primary prevention of cardiovascular events: a systematic evidence review for the U.S. Preventive Services Task Force. Evidence Synthesis No. 131. Rockville, MD: Agency for Healthcare Research and Quality; 2015.
36. Baigent C, Keech A, Kearney PM, et al. Efficacy and safety of cholesterol-lowering treatment: prospective meta-analysis of data from 90,056 participants in 14 randomised trials of statins. Lancet 2005;366:1267–78.
37. Khan AR, Riaz M, Bin Abdulhak AA, et al. The role of statins in prevention and treatment of community acquired pneumonia: a systematic review and meta-analysis. PLoS One 2013;8:e52929.
38. Chalmers JD, Singanayagam A, Murray MP, Hill AT. Prior statin use is associated with improved outcomes in community-acquired pneumonia. Am J Med 2008;121:1002–7 e1.
39. Master AM, Romanoff A, Jaffe H. Electrocardiographic changes in pneumonia. Am Heart J 1931;6:696–709.
40. Corrales-Medina VF, Musher DM, Shachkina S, Chirinos JA. Acute pneumonia and the cardiovascular system. Lancet 2015;381:496–505.
From the Division of Infectious Diseases, School of Medicine, University of Louisville, Louisville, KY.
Abstract
- Objective: To summarize the published literature on cardiac complications in patients with community-acquired pneumonia (CAP) as well as provide a historical context for the topic; and to provide recommendations concerning preventing and anticipating cardiac complications in patients with CAP.
- Methods: Literature review.
- Results: CAP patients are at increased risk for arrhythmias (~5%), myocardial infarction (~5%), and congestive heart failure (~14%). Oxygenation, the level of heart conditioning, local (pulmonary) and systemic (cytokines) inflammation, and medication all contribute to the pathophysiology of cardiac complications in CAP patients. A high Pneumonia Severity Index (PSI) can be used to screen for risk of cardiac complications in CAP patients; however, a new but less studied clinical rule developed to risk stratify patient hospitalized for CAP was shown to outperform the PSI. A troponin test and ECG should be obtained in all patients admitted for CAP while a cardiac echocardiogram may be reserved for higher-risk patients.
- Conclusions: Cardiac complications, including arrhythmias, myocardial infarctions, and congestive heart failure, are a significant burden among patients hospitalized for CAP. Influenza and pneumococcal vaccination should be emphasized among appropriate patients. Preliminary data suggest that those with CAP may be helped if they are already on aspirin or a statin. Early recognition of cardiac complications and treatment may improve clinical outcomes for patients with CAP.
Community-acquired pneumonia (CAP) is a common condition in the United States and a leading cause of morbidity and mortality [1,2], with medical costs exceeding $10 billion in 2011 [3]. The mortality rate is much higher for those aged 65 years and older [4]. Men have a higher death rate than women (18.6 vs. 13.9 per 100,000 population), and death rate varies based on ethnicity, with mortality rates for American Indian/Alaska natives at 19.2, blacks at 17.1, whites at 15.9, Asian/Pacific Islanders at 15.0, and Hispanics at 13.1 (all rates per 100,000) [2]. CAP causes considerable worldwide mortality, with differences in mortality varying according to world region [5].
Cardiovascular complications and death from other comorbidities cause a substantial proportion of CAP-associated mortality. In Mortensen et al’s study, among patients with CAP who died, at least one third had a cardiac complication, and 13% had a cardiac-related cause of death [6]. One study showed that hospitalized patients with CAP complicated by heart disease were 30% more likely to die than patients hospitalized with CAP alone [7]. In this article, we discuss the burden of cardiac complications in adults with CAP, including underlying pathophysiological processes and strategies to prevent their occurence.
Pathophysiological Processes of Heart Disease Caused by CAP
The pathophysiology of cardiac complications as a result of CAP is made up of several hypotheses, including (1) declining oxygen provision by the lungs in the face of increasing demand by the heart, (2) a lack of reserve for stress because of cardiac comorbidities and (3) localized (pulmonary) inflammation leading to systemic (including cardiac) complications by the release of cytokines or other chemicals. Any of these may result in cardiac complications occurring before, during, or after a patient has been hospitalized for CAP. Antimicrobial treatment, specifically azithromycin, has also been implicated in myocardial adverse effects. Although azithromycin is most noted for causing QT prolongation, it was associated with myocardial infarction (MI) in a study of 73,690 patients with pneumonia [8]. A higher proportion of those who received azithromycin had an MI compared to those who did not (5.1% vs 4.4%; OR 1.17; 95% CI, 1.08–1.25), but there was no statistical difference in cardiac arrhythmias, and the 90-day mortality was actually better in the azithromycin group (17.4% vs 22.3%; odds ratio [OR], 0.73; 95% CI, 0.70–0.76).
Systemic inflammation is the result of several molecules, such as cytokines, chemokines and reactive oxidant species. Reactive oxidant species may determine oxidation of proteins, lipids and DNA, which leads to cell death. The hypothesis also purports that they also cause destabilization of atherosclerotic plaques leading to MIs. Other reactions as a result of inflammation lead to arrhythmias with or without compromised cardiac function, causing congestive heart failure (CHF). For this reason, some authors have approached the pathophysiology of cardiac complications by considering them to be either plaque-related or plaque-unrelated events [9].
A few studies have linked specific inflammatory molecules to cardiac toxicity. NOX2 is chemically unstable and may provoke cellular damage, thus maintaining a certain redox balance is crucial for cardiomyocyte health. In 248 patients with CAP, an elevated troponin T was present in 135 patients and among those, NOX2 correlated with the troponin T values (OR 1.13, 95% CI 1.08–1.17; P < 0.001) [10]. Both disrupting the equilibrium of the redox balance by upregulating NOX2, and finding NOX2 to be associated with troponin T suggest that oxidative stress is implicated in damage to the myocardium during CAP. In another study of 432 patients with CAP, 41 developed atrial fibrillation within 24 to 72 hours of admission and showed higher blood levels of NOX2 than those who had CAP without atrial fibrillation [11]. Oxidative stress has been shown to cause hypertrophy, dysfunction, apoptotic cell death, and fibrosis in the myocardium [12].
There is likely a high level of variability in how individual patients respond to a predisposing factor for a cardiac complication. For example, one patient may tolerate a mild hypoxia while another is sensitive. The association of inflammatory markers with the presence of cardiac markers, however, would support that once there are systemic reactions, the complications increase. Macrolides, however, were not found to contribute to long-term mortality due to cardiac complications.
Cardiac Complications of CAP
After the H1N1 influenza outbreak of 1918, it was noted that all-cause mortality increased during the outbreak as did influenza-related deaths. This prompted inquiry as to whether there was an actual association between the outbreak and increased overall mortality, or whether the 2 occurrences were simply coincidental [14]. Near that time, arrhythmias in CAP patients were studied. T-wave changes were found to be associated with CAP [15]. Among 92 patients studied, 449 electrocardiograms (ECGs) were reviewed. T-wave changes were the most common ECG changes. They were found in 5 of 10 of the patients who died, and in 35 of the 82 patients who lived. Twelve living patients had persistent ECG changes, and although they were all thought to have had underlying myocardial disease, 2 of them certainly did as they each had an acute MI (and the ECG was included as a figure for one of them).
A study in the 1980s that reported 3 of 38 CAP patients with CHF interrupted the paucity of data at the time that showed that having a cardiac complication during CAP was a known entity [16]. By the end of the 20th century, Meier et al noted that among case patients who had an MI, an acute respiratory tract infection preceded the MI in 2.8% while in only 0.9% of control patients [17]. They also noted that patients who had an acute respiratory tract infection were 2.7 times more likely to have an MI in the following 10 days than control patients.
Further study by Musher et al revealed that MI was associated with pneumococcal pneumonia in 12 (7%) of 170 veteran patients [18]. An MI was defined on the basis of ECG abnormalities (Q waves or ST segment elevation or depression) with troponin I levels ≥ 0.5 ng/mL. They also evaluated arrhythmias and CHF. They included atrial fibrillation or flutter and ventricular tachycardia while excluding terminal arrhythmias. An arrhythmia was found in 8 (5%) patients. CHF was based on Framingham criteria (Table 1) [19]. New or worsening CHF was determined by comparing physical findings, laboratory values, chest radiograph, and echocardiogram reports in medical records. CHF was found in 13 (19%) patients. Ramirez et al found that MI was associated with CAP in 29 (5.8%) of 500 similar veteran patients [20].
Corrales-Medina et al reported cardiac complications in CAP patients in the Pneumonia Patient Outcomes Team cohort study [21]. They defined MI as the presence of 2 of 3 criteria: ECG abnormalities, elevated cardiac enzymes, and chest pain. They found 43 (3.2%) of 1343 patients with an MI. Arrhythmias included atrial fibrillation or flutter, multifocal atrial tachycardia, supraventricular tachycardia, ventricular tachycardia (≥ 3 beat run) or ventricular fibrillation. With the more inclusive list, they found a greater proportion, 137 (10%) patients affected. They defined CHF with physical examination findings plus a radiographic abnormality, and found 279 (21%) patients affected. A meta-analysis of 17 studies had pooled incidences for an MI of 5.3%, an arrhythmia of 4.7% and CHF of 14.1% [22].
In summary, the most prominent cardiac complications in patients with CAP have been found to be CHF, MI, and arrhythmia.
Timing of Cardiac Complications in Relation to CAP
While a patient is still in the community, cardiac complications may occur with the onset of CAP, or afterwards. For these patients, the primary goal is to identify the complication and manage it as soon as the patient is admitted for CAP, rather than allowing the complications to worsen only to be recognized later. Cardiac complications are rare in outpatients overall. A study of 944 outpatients found heart failure in 1.4%, arrhythmias in 1.0% and MI in 0.1% [21].
For patients who are admitted with CAP but who do not have a cardiac complication, the goals are either to prevent any complication or to recognize and manage a complication early. This also applies to patients who have been discharged after an admission for CAP. Cardiac complications have been recorded shortly after (within 30 days), and late (up to 1 year) after discharge. A study of over 50,000 veterans who were admitted for CAP were followed for any cardiovascular complication in the next 90 days. Approximately 7500 veterans were found to have a cardiac complication, including (in order of highest to lowest frequency) CHF, arrhythmia, MI, stroke and angina [23]. More than 75% of the complications were found on the day of hospitalization, but events were still measured at 30 days and 90 days.
Two other studies sought to determine an association between CAP and cardiac complications differently; not by following CAP patients prospectively for complications but by retrospectively evaluating patients for a respiratory infection among those who were admitted for a cardiovascular complication (MI or stroke). A study of over 35,000 first-time admissions for either an MI or a stroke were evaluated for a respiratory infection within the previous 90 days [24]. The incidence rates were statistically significant for every time period up to 90 days. The preceding 3 days was the time period with the highest frequency for a respiratory infection preceding an event. When the event was an MI, the incident rate was 4.95 (95% CI, 4.43–5.53). A similar study of over 20,000 first-time admissions for either an MI or stroke were evaluated for a preceding primary care visit for a respiratory infection [25]. An infection preceded 2.9% of patients with an MI and 2.8% of patients with a stroke. Statistical significance was found for the group of patients who had a respiratory infection within 7 days preceding an MI (OR 2.10 [95% CI 1.38–3.21]) or preceding a stroke (OR 1.92 [95% CI 1.24–2.97]). In fact, every time period analyzed for both complications (MI and stroke) was significant up to 1 year. Because the timing of a cardiac complication varies and can occur up to 90 days or even a year after acute infection, physicians should maintain vigilance in suspecting and screening for them.
Predictors of Cardiac Complications During CAP
Recently, Cangemi et al reviewed mortality in 301 patients admitted for CAP 6 to 60 months after they were discharged [26]. Mortality was compared between patients who experienced a cardiac complication—atrial fibrillation or an ST- or non-ST-elevation MI—during their admission and those who did not. A total of 55 (18%) patients had a cardiac complication while hospitalized. During the follow-up, 90 (30%) of the 301 patients died. Death occurred in more patients who had had a cardiac complication while hospitalized than in those who did not (32% vs 13%; P < 0.001). The study also showed that age and the pneumonia severity index (PSI) predicted death in addition to intra-hospital complication. A Cox regression analysis showed that intrahospital cardiac complications (hazard ratio [HR] 1.76 [95% CI 1.10–2.82]; P = 0.019), age (HR 1.05 [95% CI 1.03–1.08]; P < 0.001) and the PSI (HR 1.01 [95% CI 1.00–1.02] P = 0.012) independently predicted death after adjusting for possible confounders [26].
The PSI score was published in 1997, and it instructed that patients with a risk class of I or II (low risk) should be managed as outpatients. Data eventually showed that there is a portion of the population with a risk class of I or II whose hospital admission is justified [4]. Among the reasons found was “comorbidity,” including MI and other cardiac complications. The PSI prediction rule was found to be useful in novel ways, and being associated with a risk of MI in patients with CAP was one of them. The propensity-adjusted association between the PSI score and MI was significant (P < 0.05) in an observational study of the CAP Organization (CAPO) [20]. Knowing that a PSI of 80 is in the middle of risk class III (71–90), it was noted that below 80 the risk for MI was zero to 2.5%, while above 80 the risk rose from 2.5% to 12.5%. A later study using the same statistical method showed a correlation between the PSI score and cardiac complications (MI, arrhythmias and CHF) with a P value of < 0.01 [21]. Determining the probability for the combination of complications, rather than just an MI, yielded an unsurprisingly higher range of risk for the PSI below 80, which was zero to 17.5%, while risk for a PSI above 80 was 17.5% to 80%.
In a study to determine risk factors for cardiac complications among 3068 patients with CAP, Griffin et al applied a purposeful selection algorithm to a list of factors with reasonable potential to be associated with the 376 patients who actually had a cardiac complication [27]. After multivariate logistic regression analysis, hyperlipidemia, an infection with Staphlococcus aureus or Klebsiella pneumoniae, and the PSI were found to be statistically significant. In contrast, statin therapy was associated with a lower risk of an event.
In 2014, a validated score similar to the PSI and using the same database was derived to predict short-term risk for cardiac events in hospitalized patients with CAP [28]. It attributes points for age, 3 preexisting conditions, 2 vital signs and 7 radiological and laboratory values, with a point scoring system that defines 4 risk stratification classes. In the derivation cohort, the incidence of cardiac complications across the risk classes increased linearly (3%, 18%, 35%, and 72%, respectively). The score was validated in the original publication with a separate database but has not been evaluated since. The score outperformed the PSI score in predicting cardiac complications in the validation cohort (proportion of patients correctly reclassified by the new score, 44%). Potentially, the rule could help identify high-risk patients upon admission and could assist clinicians in their decision making.
Strategies to Prevent Cardiac Complications During CAP
It is now well established that there is a heavy burden of long-lasting cardiac complications among patients with CAP; therefore, preventing CAP should be a priority. This can be accomplished by counseling patients to refrain from alcohol and smoking and by administering influenza (Table 2) and pneumococcal vaccines (Figure 2). Since the 7-valent protein-polysaccharide conjugate pneumococcal vaccine (PCV-7) was released for children in 2000, there have been fewer hospitalizations in the United States [27] and improved outcomes globally;
for instance, fewer hospitalizations among children < 14 years of age in Uruguay [29], and decreased invasive pneumococcal disease among children < 5 years of age in Taiwan [30]. Furthermore, a decrease in invasive pneumococcal disease by 18% in persons aged > 65 years in the US and Canada decreased with the introduction of PCV-7 to children. Although this showed a beneficial indirect effect (herd immunity) in unvaccinated populations [31,32], there have been no randomized controlled trials in adults demonstrating a decrease in pneumococcal pneumonia or invasive pneumococcal disease which were vaccinated with PCV-13. The Food and Drug Administration approved PCV-13 for children in 2010 and for adults in 2012. Although it included fewer serotypes, it did include serotype 6A, which has a high pathogenicity and is not in 23-valent pneumococcal polysaccharide vaccine (PPSV-23). The criteria for vaccinating adults for pneumococcal infection were recently published [33]. A study of patients with invasive pneumococcal disease, which also determined pneumococcal serotypes, included 5 patients who had CAP as well [34]. Those patients had serotypes 6A, 7C, 14, and 23F (2 patients). The patient who had serotype 14 (higher pathogenicity) died and the other 4 lived. Serotypes 14 and 23F are in both vaccines while serotype 7C is in neither. Vaccination status was not provided in the study. At this time, there is evidence to support vaccinating patients for both S. pneumoniae and influenza virus.
Two methods used to prevent cardiac complications in general have been administration of aspirin and statins. The anticlotting properties of aspirin help to maintain blood flow in arteries narrowed by atherosclerosis. A meta-analysis of 10 randomized controlled trials found a statistically significant association between aspirin and a benefit on nonfatal myocardial infarctions/coronary events [35]. The associations were found with doses of 100 mg or less daily, and benefits were seen within 1 to 5 years. Statins have also been found to reduce all-cause mortality, cardiac-related mortality, and myocardial infarction [36]. A statin may stabilize coronary artery plaques that otherwise may rupture and cause myocardial ischemia or an infarct. But statins have also been found to be associated with a decreased risk of CAP. A comprehensive systematic review and meta-analysis found a decreased risk of CAP (OR 0.84; 95% CI, 0.74– 0.95) and decreased short-term mortality in patients with CAP (OR 0.68; 95% CI, 0.56–0.78) as a result of statin therapy [37]. The studies included any of 8 available statins. A prospective observational study found that patients who had been on a statin prior to being admitted for CAP had lower mortality, a lower incidence of complicated pneumonia and a lower C-reactive protein [38]. The lower C-reactive protein identifies decreased inflammation, which translates into improved endothelial function, modulated antioxidant effects, and a reduction in pro-inflammatory cytokines, hence its association with less severe CAP. Further study may reveal that a certain patient population should receive a statin to prevent CAP and improve outcomes. Overall, data support taking aspirin to prevent cardiac events regardless of CAP; further investigation of the benefits of statins to prevent cardiac complications in CAP patients is needed.
Clinical Applications
There are several implications of knowing the relationship between cardiac complications and CAP. First, physicians can better inform their patients about risks once they have been diagnosed with pneumonia. Second, physicians may be more likely to recognize a complication early and provide appropriate intervention. Third, physicians can risk stratify patients using the prediction score for cardiac complications in CAP patients [28]. In 1931 Master et al found that some patients with CAP also had PR interval or T-wave changes present for about 3 days, so they recommended obtaining an ECG to determine when a patient might be able to be discharged or declared “cured” [39]. Now, we are similarly recommending obtaining an ECG in CAP patients, but upon admission, in order to identify those who may get ischemic changes, arrhythmias or QTc prolongations. Pro-brain natriuretic peptide and troponins may be obtained independently of ECG results, and a cardiac echocardiogram may be reserved for those with a high risk of complications [40]. Finally, we recommend screening all patients for need for influenza and pneumococcal vaccines and administering according to the Advisory Committee on Immunization Practices of the Centers for Disease and Prevention [33].
Research Implications
The fact that cardiac complications in CAP patients is a well-defined entity with a significant degree of morbidity and mortality should prompt attentiona and resources to be directed to this area. The prediction score created specifically for this subpopulation of patients [28] can improve research by allowing adequate risk stratification to efficiently design and execute studies. Studies may be designed with fewer patients required to be enrolled while maintaining statistical power by limiting subject inclusion criteria to certain risk classes. Specific areas of future investigation should include the mechanisms of pathophysiology, which are not completely understood, and other complications, such as pulmonary edema, infectious endocarditis and pericarditis. Finally, cost has not been studied in this area or the potential savings of recognizing and preventing cardiac complications.
Summary
Cardiac complications, including arrhythmias, MI, and CHF are a significant burden among patients hospitalized for CAP. Influenza and pneumococcal vaccination should be emphasized among appropriate patients. The cardiac complication prediction score may be used to screen patients once admitted. A troponin and ECG should be obtained in all patients admitted for CAP while a cardiac echocardiogram may be reserved in higher-risk patients. Future research may be directed towards the subjects of pathophysiology other complications and cost.
Acknowledgment: We appreciate the critical review by Jessica Lynn Petrey, MSLS, Clinical Librarian, Kornhauser Health Sciences Library, University of Louisville, Louisville, KY.
Corresponding author: Dr. Forest Arnold, 501 E. Broadway, Suite 140 B, Louisville, KY 40202, f.arnold@louisville.edu
Financial disclosures: None.
From the Division of Infectious Diseases, School of Medicine, University of Louisville, Louisville, KY.
Abstract
- Objective: To summarize the published literature on cardiac complications in patients with community-acquired pneumonia (CAP) as well as provide a historical context for the topic; and to provide recommendations concerning preventing and anticipating cardiac complications in patients with CAP.
- Methods: Literature review.
- Results: CAP patients are at increased risk for arrhythmias (~5%), myocardial infarction (~5%), and congestive heart failure (~14%). Oxygenation, the level of heart conditioning, local (pulmonary) and systemic (cytokines) inflammation, and medication all contribute to the pathophysiology of cardiac complications in CAP patients. A high Pneumonia Severity Index (PSI) can be used to screen for risk of cardiac complications in CAP patients; however, a new but less studied clinical rule developed to risk stratify patient hospitalized for CAP was shown to outperform the PSI. A troponin test and ECG should be obtained in all patients admitted for CAP while a cardiac echocardiogram may be reserved for higher-risk patients.
- Conclusions: Cardiac complications, including arrhythmias, myocardial infarctions, and congestive heart failure, are a significant burden among patients hospitalized for CAP. Influenza and pneumococcal vaccination should be emphasized among appropriate patients. Preliminary data suggest that those with CAP may be helped if they are already on aspirin or a statin. Early recognition of cardiac complications and treatment may improve clinical outcomes for patients with CAP.
Community-acquired pneumonia (CAP) is a common condition in the United States and a leading cause of morbidity and mortality [1,2], with medical costs exceeding $10 billion in 2011 [3]. The mortality rate is much higher for those aged 65 years and older [4]. Men have a higher death rate than women (18.6 vs. 13.9 per 100,000 population), and death rate varies based on ethnicity, with mortality rates for American Indian/Alaska natives at 19.2, blacks at 17.1, whites at 15.9, Asian/Pacific Islanders at 15.0, and Hispanics at 13.1 (all rates per 100,000) [2]. CAP causes considerable worldwide mortality, with differences in mortality varying according to world region [5].
Cardiovascular complications and death from other comorbidities cause a substantial proportion of CAP-associated mortality. In Mortensen et al’s study, among patients with CAP who died, at least one third had a cardiac complication, and 13% had a cardiac-related cause of death [6]. One study showed that hospitalized patients with CAP complicated by heart disease were 30% more likely to die than patients hospitalized with CAP alone [7]. In this article, we discuss the burden of cardiac complications in adults with CAP, including underlying pathophysiological processes and strategies to prevent their occurence.
Pathophysiological Processes of Heart Disease Caused by CAP
The pathophysiology of cardiac complications as a result of CAP is made up of several hypotheses, including (1) declining oxygen provision by the lungs in the face of increasing demand by the heart, (2) a lack of reserve for stress because of cardiac comorbidities and (3) localized (pulmonary) inflammation leading to systemic (including cardiac) complications by the release of cytokines or other chemicals. Any of these may result in cardiac complications occurring before, during, or after a patient has been hospitalized for CAP. Antimicrobial treatment, specifically azithromycin, has also been implicated in myocardial adverse effects. Although azithromycin is most noted for causing QT prolongation, it was associated with myocardial infarction (MI) in a study of 73,690 patients with pneumonia [8]. A higher proportion of those who received azithromycin had an MI compared to those who did not (5.1% vs 4.4%; OR 1.17; 95% CI, 1.08–1.25), but there was no statistical difference in cardiac arrhythmias, and the 90-day mortality was actually better in the azithromycin group (17.4% vs 22.3%; odds ratio [OR], 0.73; 95% CI, 0.70–0.76).
Systemic inflammation is the result of several molecules, such as cytokines, chemokines and reactive oxidant species. Reactive oxidant species may determine oxidation of proteins, lipids and DNA, which leads to cell death. The hypothesis also purports that they also cause destabilization of atherosclerotic plaques leading to MIs. Other reactions as a result of inflammation lead to arrhythmias with or without compromised cardiac function, causing congestive heart failure (CHF). For this reason, some authors have approached the pathophysiology of cardiac complications by considering them to be either plaque-related or plaque-unrelated events [9].
A few studies have linked specific inflammatory molecules to cardiac toxicity. NOX2 is chemically unstable and may provoke cellular damage, thus maintaining a certain redox balance is crucial for cardiomyocyte health. In 248 patients with CAP, an elevated troponin T was present in 135 patients and among those, NOX2 correlated with the troponin T values (OR 1.13, 95% CI 1.08–1.17; P < 0.001) [10]. Both disrupting the equilibrium of the redox balance by upregulating NOX2, and finding NOX2 to be associated with troponin T suggest that oxidative stress is implicated in damage to the myocardium during CAP. In another study of 432 patients with CAP, 41 developed atrial fibrillation within 24 to 72 hours of admission and showed higher blood levels of NOX2 than those who had CAP without atrial fibrillation [11]. Oxidative stress has been shown to cause hypertrophy, dysfunction, apoptotic cell death, and fibrosis in the myocardium [12].
There is likely a high level of variability in how individual patients respond to a predisposing factor for a cardiac complication. For example, one patient may tolerate a mild hypoxia while another is sensitive. The association of inflammatory markers with the presence of cardiac markers, however, would support that once there are systemic reactions, the complications increase. Macrolides, however, were not found to contribute to long-term mortality due to cardiac complications.
Cardiac Complications of CAP
After the H1N1 influenza outbreak of 1918, it was noted that all-cause mortality increased during the outbreak as did influenza-related deaths. This prompted inquiry as to whether there was an actual association between the outbreak and increased overall mortality, or whether the 2 occurrences were simply coincidental [14]. Near that time, arrhythmias in CAP patients were studied. T-wave changes were found to be associated with CAP [15]. Among 92 patients studied, 449 electrocardiograms (ECGs) were reviewed. T-wave changes were the most common ECG changes. They were found in 5 of 10 of the patients who died, and in 35 of the 82 patients who lived. Twelve living patients had persistent ECG changes, and although they were all thought to have had underlying myocardial disease, 2 of them certainly did as they each had an acute MI (and the ECG was included as a figure for one of them).
A study in the 1980s that reported 3 of 38 CAP patients with CHF interrupted the paucity of data at the time that showed that having a cardiac complication during CAP was a known entity [16]. By the end of the 20th century, Meier et al noted that among case patients who had an MI, an acute respiratory tract infection preceded the MI in 2.8% while in only 0.9% of control patients [17]. They also noted that patients who had an acute respiratory tract infection were 2.7 times more likely to have an MI in the following 10 days than control patients.
Further study by Musher et al revealed that MI was associated with pneumococcal pneumonia in 12 (7%) of 170 veteran patients [18]. An MI was defined on the basis of ECG abnormalities (Q waves or ST segment elevation or depression) with troponin I levels ≥ 0.5 ng/mL. They also evaluated arrhythmias and CHF. They included atrial fibrillation or flutter and ventricular tachycardia while excluding terminal arrhythmias. An arrhythmia was found in 8 (5%) patients. CHF was based on Framingham criteria (Table 1) [19]. New or worsening CHF was determined by comparing physical findings, laboratory values, chest radiograph, and echocardiogram reports in medical records. CHF was found in 13 (19%) patients. Ramirez et al found that MI was associated with CAP in 29 (5.8%) of 500 similar veteran patients [20].
Corrales-Medina et al reported cardiac complications in CAP patients in the Pneumonia Patient Outcomes Team cohort study [21]. They defined MI as the presence of 2 of 3 criteria: ECG abnormalities, elevated cardiac enzymes, and chest pain. They found 43 (3.2%) of 1343 patients with an MI. Arrhythmias included atrial fibrillation or flutter, multifocal atrial tachycardia, supraventricular tachycardia, ventricular tachycardia (≥ 3 beat run) or ventricular fibrillation. With the more inclusive list, they found a greater proportion, 137 (10%) patients affected. They defined CHF with physical examination findings plus a radiographic abnormality, and found 279 (21%) patients affected. A meta-analysis of 17 studies had pooled incidences for an MI of 5.3%, an arrhythmia of 4.7% and CHF of 14.1% [22].
In summary, the most prominent cardiac complications in patients with CAP have been found to be CHF, MI, and arrhythmia.
Timing of Cardiac Complications in Relation to CAP
While a patient is still in the community, cardiac complications may occur with the onset of CAP, or afterwards. For these patients, the primary goal is to identify the complication and manage it as soon as the patient is admitted for CAP, rather than allowing the complications to worsen only to be recognized later. Cardiac complications are rare in outpatients overall. A study of 944 outpatients found heart failure in 1.4%, arrhythmias in 1.0% and MI in 0.1% [21].
For patients who are admitted with CAP but who do not have a cardiac complication, the goals are either to prevent any complication or to recognize and manage a complication early. This also applies to patients who have been discharged after an admission for CAP. Cardiac complications have been recorded shortly after (within 30 days), and late (up to 1 year) after discharge. A study of over 50,000 veterans who were admitted for CAP were followed for any cardiovascular complication in the next 90 days. Approximately 7500 veterans were found to have a cardiac complication, including (in order of highest to lowest frequency) CHF, arrhythmia, MI, stroke and angina [23]. More than 75% of the complications were found on the day of hospitalization, but events were still measured at 30 days and 90 days.
Two other studies sought to determine an association between CAP and cardiac complications differently; not by following CAP patients prospectively for complications but by retrospectively evaluating patients for a respiratory infection among those who were admitted for a cardiovascular complication (MI or stroke). A study of over 35,000 first-time admissions for either an MI or a stroke were evaluated for a respiratory infection within the previous 90 days [24]. The incidence rates were statistically significant for every time period up to 90 days. The preceding 3 days was the time period with the highest frequency for a respiratory infection preceding an event. When the event was an MI, the incident rate was 4.95 (95% CI, 4.43–5.53). A similar study of over 20,000 first-time admissions for either an MI or stroke were evaluated for a preceding primary care visit for a respiratory infection [25]. An infection preceded 2.9% of patients with an MI and 2.8% of patients with a stroke. Statistical significance was found for the group of patients who had a respiratory infection within 7 days preceding an MI (OR 2.10 [95% CI 1.38–3.21]) or preceding a stroke (OR 1.92 [95% CI 1.24–2.97]). In fact, every time period analyzed for both complications (MI and stroke) was significant up to 1 year. Because the timing of a cardiac complication varies and can occur up to 90 days or even a year after acute infection, physicians should maintain vigilance in suspecting and screening for them.
Predictors of Cardiac Complications During CAP
Recently, Cangemi et al reviewed mortality in 301 patients admitted for CAP 6 to 60 months after they were discharged [26]. Mortality was compared between patients who experienced a cardiac complication—atrial fibrillation or an ST- or non-ST-elevation MI—during their admission and those who did not. A total of 55 (18%) patients had a cardiac complication while hospitalized. During the follow-up, 90 (30%) of the 301 patients died. Death occurred in more patients who had had a cardiac complication while hospitalized than in those who did not (32% vs 13%; P < 0.001). The study also showed that age and the pneumonia severity index (PSI) predicted death in addition to intra-hospital complication. A Cox regression analysis showed that intrahospital cardiac complications (hazard ratio [HR] 1.76 [95% CI 1.10–2.82]; P = 0.019), age (HR 1.05 [95% CI 1.03–1.08]; P < 0.001) and the PSI (HR 1.01 [95% CI 1.00–1.02] P = 0.012) independently predicted death after adjusting for possible confounders [26].
The PSI score was published in 1997, and it instructed that patients with a risk class of I or II (low risk) should be managed as outpatients. Data eventually showed that there is a portion of the population with a risk class of I or II whose hospital admission is justified [4]. Among the reasons found was “comorbidity,” including MI and other cardiac complications. The PSI prediction rule was found to be useful in novel ways, and being associated with a risk of MI in patients with CAP was one of them. The propensity-adjusted association between the PSI score and MI was significant (P < 0.05) in an observational study of the CAP Organization (CAPO) [20]. Knowing that a PSI of 80 is in the middle of risk class III (71–90), it was noted that below 80 the risk for MI was zero to 2.5%, while above 80 the risk rose from 2.5% to 12.5%. A later study using the same statistical method showed a correlation between the PSI score and cardiac complications (MI, arrhythmias and CHF) with a P value of < 0.01 [21]. Determining the probability for the combination of complications, rather than just an MI, yielded an unsurprisingly higher range of risk for the PSI below 80, which was zero to 17.5%, while risk for a PSI above 80 was 17.5% to 80%.
In a study to determine risk factors for cardiac complications among 3068 patients with CAP, Griffin et al applied a purposeful selection algorithm to a list of factors with reasonable potential to be associated with the 376 patients who actually had a cardiac complication [27]. After multivariate logistic regression analysis, hyperlipidemia, an infection with Staphlococcus aureus or Klebsiella pneumoniae, and the PSI were found to be statistically significant. In contrast, statin therapy was associated with a lower risk of an event.
In 2014, a validated score similar to the PSI and using the same database was derived to predict short-term risk for cardiac events in hospitalized patients with CAP [28]. It attributes points for age, 3 preexisting conditions, 2 vital signs and 7 radiological and laboratory values, with a point scoring system that defines 4 risk stratification classes. In the derivation cohort, the incidence of cardiac complications across the risk classes increased linearly (3%, 18%, 35%, and 72%, respectively). The score was validated in the original publication with a separate database but has not been evaluated since. The score outperformed the PSI score in predicting cardiac complications in the validation cohort (proportion of patients correctly reclassified by the new score, 44%). Potentially, the rule could help identify high-risk patients upon admission and could assist clinicians in their decision making.
Strategies to Prevent Cardiac Complications During CAP
It is now well established that there is a heavy burden of long-lasting cardiac complications among patients with CAP; therefore, preventing CAP should be a priority. This can be accomplished by counseling patients to refrain from alcohol and smoking and by administering influenza (Table 2) and pneumococcal vaccines (Figure 2). Since the 7-valent protein-polysaccharide conjugate pneumococcal vaccine (PCV-7) was released for children in 2000, there have been fewer hospitalizations in the United States [27] and improved outcomes globally;
for instance, fewer hospitalizations among children < 14 years of age in Uruguay [29], and decreased invasive pneumococcal disease among children < 5 years of age in Taiwan [30]. Furthermore, a decrease in invasive pneumococcal disease by 18% in persons aged > 65 years in the US and Canada decreased with the introduction of PCV-7 to children. Although this showed a beneficial indirect effect (herd immunity) in unvaccinated populations [31,32], there have been no randomized controlled trials in adults demonstrating a decrease in pneumococcal pneumonia or invasive pneumococcal disease which were vaccinated with PCV-13. The Food and Drug Administration approved PCV-13 for children in 2010 and for adults in 2012. Although it included fewer serotypes, it did include serotype 6A, which has a high pathogenicity and is not in 23-valent pneumococcal polysaccharide vaccine (PPSV-23). The criteria for vaccinating adults for pneumococcal infection were recently published [33]. A study of patients with invasive pneumococcal disease, which also determined pneumococcal serotypes, included 5 patients who had CAP as well [34]. Those patients had serotypes 6A, 7C, 14, and 23F (2 patients). The patient who had serotype 14 (higher pathogenicity) died and the other 4 lived. Serotypes 14 and 23F are in both vaccines while serotype 7C is in neither. Vaccination status was not provided in the study. At this time, there is evidence to support vaccinating patients for both S. pneumoniae and influenza virus.
Two methods used to prevent cardiac complications in general have been administration of aspirin and statins. The anticlotting properties of aspirin help to maintain blood flow in arteries narrowed by atherosclerosis. A meta-analysis of 10 randomized controlled trials found a statistically significant association between aspirin and a benefit on nonfatal myocardial infarctions/coronary events [35]. The associations were found with doses of 100 mg or less daily, and benefits were seen within 1 to 5 years. Statins have also been found to reduce all-cause mortality, cardiac-related mortality, and myocardial infarction [36]. A statin may stabilize coronary artery plaques that otherwise may rupture and cause myocardial ischemia or an infarct. But statins have also been found to be associated with a decreased risk of CAP. A comprehensive systematic review and meta-analysis found a decreased risk of CAP (OR 0.84; 95% CI, 0.74– 0.95) and decreased short-term mortality in patients with CAP (OR 0.68; 95% CI, 0.56–0.78) as a result of statin therapy [37]. The studies included any of 8 available statins. A prospective observational study found that patients who had been on a statin prior to being admitted for CAP had lower mortality, a lower incidence of complicated pneumonia and a lower C-reactive protein [38]. The lower C-reactive protein identifies decreased inflammation, which translates into improved endothelial function, modulated antioxidant effects, and a reduction in pro-inflammatory cytokines, hence its association with less severe CAP. Further study may reveal that a certain patient population should receive a statin to prevent CAP and improve outcomes. Overall, data support taking aspirin to prevent cardiac events regardless of CAP; further investigation of the benefits of statins to prevent cardiac complications in CAP patients is needed.
Clinical Applications
There are several implications of knowing the relationship between cardiac complications and CAP. First, physicians can better inform their patients about risks once they have been diagnosed with pneumonia. Second, physicians may be more likely to recognize a complication early and provide appropriate intervention. Third, physicians can risk stratify patients using the prediction score for cardiac complications in CAP patients [28]. In 1931 Master et al found that some patients with CAP also had PR interval or T-wave changes present for about 3 days, so they recommended obtaining an ECG to determine when a patient might be able to be discharged or declared “cured” [39]. Now, we are similarly recommending obtaining an ECG in CAP patients, but upon admission, in order to identify those who may get ischemic changes, arrhythmias or QTc prolongations. Pro-brain natriuretic peptide and troponins may be obtained independently of ECG results, and a cardiac echocardiogram may be reserved for those with a high risk of complications [40]. Finally, we recommend screening all patients for need for influenza and pneumococcal vaccines and administering according to the Advisory Committee on Immunization Practices of the Centers for Disease and Prevention [33].
Research Implications
The fact that cardiac complications in CAP patients is a well-defined entity with a significant degree of morbidity and mortality should prompt attentiona and resources to be directed to this area. The prediction score created specifically for this subpopulation of patients [28] can improve research by allowing adequate risk stratification to efficiently design and execute studies. Studies may be designed with fewer patients required to be enrolled while maintaining statistical power by limiting subject inclusion criteria to certain risk classes. Specific areas of future investigation should include the mechanisms of pathophysiology, which are not completely understood, and other complications, such as pulmonary edema, infectious endocarditis and pericarditis. Finally, cost has not been studied in this area or the potential savings of recognizing and preventing cardiac complications.
Summary
Cardiac complications, including arrhythmias, MI, and CHF are a significant burden among patients hospitalized for CAP. Influenza and pneumococcal vaccination should be emphasized among appropriate patients. The cardiac complication prediction score may be used to screen patients once admitted. A troponin and ECG should be obtained in all patients admitted for CAP while a cardiac echocardiogram may be reserved in higher-risk patients. Future research may be directed towards the subjects of pathophysiology other complications and cost.
Acknowledgment: We appreciate the critical review by Jessica Lynn Petrey, MSLS, Clinical Librarian, Kornhauser Health Sciences Library, University of Louisville, Louisville, KY.
Corresponding author: Dr. Forest Arnold, 501 E. Broadway, Suite 140 B, Louisville, KY 40202, f.arnold@louisville.edu
Financial disclosures: None.
1. Pfuntner A, Wier LM, Stocks C. HCUP statistical brief #162. Agency for Healthcare Research and Quality; Rockville, MD: 2013. Most frequent conditions in U.S. hospitals, 2011. Available at www.hcup-us.ahrq.gov/reports/statbriefs/sb162.pdf..
2. FastStats deaths and mortality. Centers for Disease Control and Prevention. Accessed 14 Oct 2015 at www.cdc.gov/nchs/fastats/deaths.htm.
3. Pfuntner A, Wier LM, Steiner C. HCUP statistical brief #168. Agency for Healthcare Research and Quality; Rockville, MD: 2013. Costs for hospital stays in the United States, 2011. Available at www.hcup-us.ahrq.gov/reports/statbriefs/sb168-Hospital-Costs-United-States-2011.pdf.
4. American Lung Association. Trends in pneumonia and influenza morbidity and mortality. November 2015. Available at
www.lung.org/assets/documents/research/pi-trend-report.pdf.
5. Arnold FW, Wiemken TL, Peyrani P, et al. Mortality differences among hospitalized patients with community-acquired pneumonia in three world regions: results from the Community-Acquired Pneumonia Organization (CAPO) International Cohort Study. Respir Med 2013;107:1101–11.
6. Mortensen EM, Coley CM, Singer DE, et al. Causes of death for patients with community-acquired pneumonia: results from the Pneumonia Patient Outcomes Research Team cohort study. Arch Intern Med 2002;162:1059–64.
7. Bordon J, Wiemken T, Peyrani P, et al. Decrease in long-term survival for hospitalized patients with community-acquired pneumonia. Chest 2010;138:279–83.
8. Mortensen EM, Halm EA, Pugh MJ, et al. Association of azithromycin with mortality and cardiovascular events among older patients hospitalized with pneumonia. JAMA 2014;311:2199–208.
9. Aliberti S, Ramirez JA. Cardiac diseases complicating community-acquired pneumonia. Curr Opin Infect Dis 2014;27:295–301.
10. Cangemi R, Calvieri C, Bucci T, et al. Is NOX2 upregulation implicated in myocardial injury in patients with pneumonia? Antioxid Redox Signal 2014;20:2949–54.
11. Violi F, Carnevale R, Calvieri C, et al. Nox2 up-regulation is associated with an enhanced risk of atrial fibrillation in patients with pneumonia. Thorax 2015;70:961–6.
12. Zhang Y, Tocchetti CG, Krieg T, Moens AL. Oxidative and nitrosative stress in the maintenance of myocardial function. Free Radic Biol Med 2012;53:1531–40.
13. Brown AO, Millett ER, Quint JK, Orihuela CJ. Cardiotoxicity during invasive pneumococcal disease. Am J Respir Crit Care Med 2015;191:739–45.
14. Collins SD. Excess mortality from causes other than influenza and pneumonia during influenza epidemics. Pub Health Rep 1932;47:2159–79.
15. Thomson KJ, Rustein DD, et al. Electrocardiographic studies during and after pneumococcus pneumonia. Am Heart J 1946;31:565–79.
16. Esposito AL. Community-acquired bacteremic pneumococcal pneumonia. Effect of age on manifestations and outcome. Arch Intern Med 1984;144:945–8.
17. Meier CR, Jick SS, Derby LE, et al. Acute respiratory-tract infections and risk of first-time acute myocardial infarction. Lancet 1998;351(9114):1467–71.
18. Musher DM, Rueda AM, Kaka AS, Mapara SM. The association between pneumococcal pneumonia and acute cardiac events. Clin Infect Dis 2007;45:158–65.
19. McKee PA, Castelli WP, McNamara PM, Kannel WB. The natural history of congestive heart failure: the Framingham study. N Engl J Med 1971;285:1441–6.
20. Ramirez J, Aliberti S, Mirsaeidi M, et al. Acute myocardial infarction in hospitalized patients with community-acquired pneumonia. Clin Infect Dis 2008;47:182–7.
21. Corrales-Medina VF, Musher DM, Wells GA, et al. Cardiac complications in patients with community-acquired pneumonia: incidence, timing, risk factors, and association with short-term mortality. Circulation 2012;125:773–81.
22. Corrales-Medina VF, Suh KN, Rose G, et al. Cardiac complications in patients with community-acquired pneumonia: a systematic review and meta-analysis of observational studies. PLoS Med 2011;8(6):e1001048.
23. Perry TW, Pugh MJ, Waterer GW, et al. Incidence of cardiovascular events after hospital admission for pneumonia. Am J Med 2011;124:244–51.
24. Smeeth L, Thomas SL, Hall AJ, et al. Risk of myocardial infarction and stroke after acute infection or vaccination. N Engl J Med 2004;351:2611–8.
25. Clayton TC, Thompson M, Meade TW. Recent respiratory infection and risk of cardiovascular disease: case-control study through a general practice database. Eur Heart J 2008;29:96–103.
26. Cangemi R, Calvieri C, Falcone M, et al. Relation of cardiac complications in the early phase of community-acquired pneumonia to long-term mortality and cardiovascular events. Am J Cardiol 2015;116:647–51.
27. Griffin MR, Zhu Y, Moore MR, et al. U.S. hospitalizations for pneumonia after a decade of pneumococcal vaccination. N Engl J Med 2013;369:155–63.
28. Corrales-Medina VF, Taljaard M, Fine MJ, et al. Risk stratification for cardiac complications in patients hospitalized for community-acquired pneumonia. Mayo Clin Proc 2014;89:60–8.
29. Pirez MC, Algorta G, Cedres A, et al. Impact of universal pneumococcal vaccination on hospitalizations for pneumonia and meningitis in children in Montevideo, Uruguay. Pediatr Infect Dis J 2011;30:669–74.
30. Liao WH, Lin SH, Lai CC, et al. Impact of pneumococcal vaccines on invasive pneumococcal disease in Taiwan. Eur J Clin Microbiol Infect Dis 2010;29:489–92.
31. Whitney CG, Farley MM, Hadler J, et al. Decline in invasive pneumococcal disease after the introduction of protein-polysaccharide conjugate vaccine. N Engl J Med 2003;348:1737–46.
32. Kellner JD, Church DL, MacDonald J, et al. Progress in the prevention of pneumococcal infection. CMAJ 2005;173:1149–51.
33. Kim DK, Bridges CB, Harriman KH, Advisory Committee on Immunization Practices. Recommended immunization schedule for adults aged 19 years or older: United States, 2016. Ann Intern Med 2016;164:184–94.
34. Kan B, Ries J, Normark BH, et al. Endocarditis and pericarditis complicating pneumococcal bacteraemia, with special reference to the adhesive abilities of pneumococci: results from a prospective study. Clin Microbiol Infect 2006;12:338–44.
35. Guirguis-Blake JM, Evans CV, Senger CA, et al. Aspirin for the primary prevention of cardiovascular events: a systematic evidence review for the U.S. Preventive Services Task Force. Evidence Synthesis No. 131. Rockville, MD: Agency for Healthcare Research and Quality; 2015.
36. Baigent C, Keech A, Kearney PM, et al. Efficacy and safety of cholesterol-lowering treatment: prospective meta-analysis of data from 90,056 participants in 14 randomised trials of statins. Lancet 2005;366:1267–78.
37. Khan AR, Riaz M, Bin Abdulhak AA, et al. The role of statins in prevention and treatment of community acquired pneumonia: a systematic review and meta-analysis. PLoS One 2013;8:e52929.
38. Chalmers JD, Singanayagam A, Murray MP, Hill AT. Prior statin use is associated with improved outcomes in community-acquired pneumonia. Am J Med 2008;121:1002–7 e1.
39. Master AM, Romanoff A, Jaffe H. Electrocardiographic changes in pneumonia. Am Heart J 1931;6:696–709.
40. Corrales-Medina VF, Musher DM, Shachkina S, Chirinos JA. Acute pneumonia and the cardiovascular system. Lancet 2015;381:496–505.
1. Pfuntner A, Wier LM, Stocks C. HCUP statistical brief #162. Agency for Healthcare Research and Quality; Rockville, MD: 2013. Most frequent conditions in U.S. hospitals, 2011. Available at www.hcup-us.ahrq.gov/reports/statbriefs/sb162.pdf..
2. FastStats deaths and mortality. Centers for Disease Control and Prevention. Accessed 14 Oct 2015 at www.cdc.gov/nchs/fastats/deaths.htm.
3. Pfuntner A, Wier LM, Steiner C. HCUP statistical brief #168. Agency for Healthcare Research and Quality; Rockville, MD: 2013. Costs for hospital stays in the United States, 2011. Available at www.hcup-us.ahrq.gov/reports/statbriefs/sb168-Hospital-Costs-United-States-2011.pdf.
4. American Lung Association. Trends in pneumonia and influenza morbidity and mortality. November 2015. Available at
www.lung.org/assets/documents/research/pi-trend-report.pdf.
5. Arnold FW, Wiemken TL, Peyrani P, et al. Mortality differences among hospitalized patients with community-acquired pneumonia in three world regions: results from the Community-Acquired Pneumonia Organization (CAPO) International Cohort Study. Respir Med 2013;107:1101–11.
6. Mortensen EM, Coley CM, Singer DE, et al. Causes of death for patients with community-acquired pneumonia: results from the Pneumonia Patient Outcomes Research Team cohort study. Arch Intern Med 2002;162:1059–64.
7. Bordon J, Wiemken T, Peyrani P, et al. Decrease in long-term survival for hospitalized patients with community-acquired pneumonia. Chest 2010;138:279–83.
8. Mortensen EM, Halm EA, Pugh MJ, et al. Association of azithromycin with mortality and cardiovascular events among older patients hospitalized with pneumonia. JAMA 2014;311:2199–208.
9. Aliberti S, Ramirez JA. Cardiac diseases complicating community-acquired pneumonia. Curr Opin Infect Dis 2014;27:295–301.
10. Cangemi R, Calvieri C, Bucci T, et al. Is NOX2 upregulation implicated in myocardial injury in patients with pneumonia? Antioxid Redox Signal 2014;20:2949–54.
11. Violi F, Carnevale R, Calvieri C, et al. Nox2 up-regulation is associated with an enhanced risk of atrial fibrillation in patients with pneumonia. Thorax 2015;70:961–6.
12. Zhang Y, Tocchetti CG, Krieg T, Moens AL. Oxidative and nitrosative stress in the maintenance of myocardial function. Free Radic Biol Med 2012;53:1531–40.
13. Brown AO, Millett ER, Quint JK, Orihuela CJ. Cardiotoxicity during invasive pneumococcal disease. Am J Respir Crit Care Med 2015;191:739–45.
14. Collins SD. Excess mortality from causes other than influenza and pneumonia during influenza epidemics. Pub Health Rep 1932;47:2159–79.
15. Thomson KJ, Rustein DD, et al. Electrocardiographic studies during and after pneumococcus pneumonia. Am Heart J 1946;31:565–79.
16. Esposito AL. Community-acquired bacteremic pneumococcal pneumonia. Effect of age on manifestations and outcome. Arch Intern Med 1984;144:945–8.
17. Meier CR, Jick SS, Derby LE, et al. Acute respiratory-tract infections and risk of first-time acute myocardial infarction. Lancet 1998;351(9114):1467–71.
18. Musher DM, Rueda AM, Kaka AS, Mapara SM. The association between pneumococcal pneumonia and acute cardiac events. Clin Infect Dis 2007;45:158–65.
19. McKee PA, Castelli WP, McNamara PM, Kannel WB. The natural history of congestive heart failure: the Framingham study. N Engl J Med 1971;285:1441–6.
20. Ramirez J, Aliberti S, Mirsaeidi M, et al. Acute myocardial infarction in hospitalized patients with community-acquired pneumonia. Clin Infect Dis 2008;47:182–7.
21. Corrales-Medina VF, Musher DM, Wells GA, et al. Cardiac complications in patients with community-acquired pneumonia: incidence, timing, risk factors, and association with short-term mortality. Circulation 2012;125:773–81.
22. Corrales-Medina VF, Suh KN, Rose G, et al. Cardiac complications in patients with community-acquired pneumonia: a systematic review and meta-analysis of observational studies. PLoS Med 2011;8(6):e1001048.
23. Perry TW, Pugh MJ, Waterer GW, et al. Incidence of cardiovascular events after hospital admission for pneumonia. Am J Med 2011;124:244–51.
24. Smeeth L, Thomas SL, Hall AJ, et al. Risk of myocardial infarction and stroke after acute infection or vaccination. N Engl J Med 2004;351:2611–8.
25. Clayton TC, Thompson M, Meade TW. Recent respiratory infection and risk of cardiovascular disease: case-control study through a general practice database. Eur Heart J 2008;29:96–103.
26. Cangemi R, Calvieri C, Falcone M, et al. Relation of cardiac complications in the early phase of community-acquired pneumonia to long-term mortality and cardiovascular events. Am J Cardiol 2015;116:647–51.
27. Griffin MR, Zhu Y, Moore MR, et al. U.S. hospitalizations for pneumonia after a decade of pneumococcal vaccination. N Engl J Med 2013;369:155–63.
28. Corrales-Medina VF, Taljaard M, Fine MJ, et al. Risk stratification for cardiac complications in patients hospitalized for community-acquired pneumonia. Mayo Clin Proc 2014;89:60–8.
29. Pirez MC, Algorta G, Cedres A, et al. Impact of universal pneumococcal vaccination on hospitalizations for pneumonia and meningitis in children in Montevideo, Uruguay. Pediatr Infect Dis J 2011;30:669–74.
30. Liao WH, Lin SH, Lai CC, et al. Impact of pneumococcal vaccines on invasive pneumococcal disease in Taiwan. Eur J Clin Microbiol Infect Dis 2010;29:489–92.
31. Whitney CG, Farley MM, Hadler J, et al. Decline in invasive pneumococcal disease after the introduction of protein-polysaccharide conjugate vaccine. N Engl J Med 2003;348:1737–46.
32. Kellner JD, Church DL, MacDonald J, et al. Progress in the prevention of pneumococcal infection. CMAJ 2005;173:1149–51.
33. Kim DK, Bridges CB, Harriman KH, Advisory Committee on Immunization Practices. Recommended immunization schedule for adults aged 19 years or older: United States, 2016. Ann Intern Med 2016;164:184–94.
34. Kan B, Ries J, Normark BH, et al. Endocarditis and pericarditis complicating pneumococcal bacteraemia, with special reference to the adhesive abilities of pneumococci: results from a prospective study. Clin Microbiol Infect 2006;12:338–44.
35. Guirguis-Blake JM, Evans CV, Senger CA, et al. Aspirin for the primary prevention of cardiovascular events: a systematic evidence review for the U.S. Preventive Services Task Force. Evidence Synthesis No. 131. Rockville, MD: Agency for Healthcare Research and Quality; 2015.
36. Baigent C, Keech A, Kearney PM, et al. Efficacy and safety of cholesterol-lowering treatment: prospective meta-analysis of data from 90,056 participants in 14 randomised trials of statins. Lancet 2005;366:1267–78.
37. Khan AR, Riaz M, Bin Abdulhak AA, et al. The role of statins in prevention and treatment of community acquired pneumonia: a systematic review and meta-analysis. PLoS One 2013;8:e52929.
38. Chalmers JD, Singanayagam A, Murray MP, Hill AT. Prior statin use is associated with improved outcomes in community-acquired pneumonia. Am J Med 2008;121:1002–7 e1.
39. Master AM, Romanoff A, Jaffe H. Electrocardiographic changes in pneumonia. Am Heart J 1931;6:696–709.
40. Corrales-Medina VF, Musher DM, Shachkina S, Chirinos JA. Acute pneumonia and the cardiovascular system. Lancet 2015;381:496–505.
CAPO Aspiration Pneumonia
Pneumonia is a common clinical syndrome with well‐described epidemiology and microbiology. Aspiration pneumonia comprises 5% to 15% of patients with pneumonia acquired outside of the hospital,[1] but is less well characterized despite being a major syndrome of pneumonia in the elderly.[2, 3] Difficulties in studying aspiration pneumonia include the lack of a sensitive and specific marker for aspiration as well as the potential overlap between aspiration pneumonia and other forms of pneumonia.[4, 5, 6] Additionally, clinicians have difficulty distinguishing between aspiration pneumonia, which develops after the aspiration of oropharyngeal contents, and aspiration pneumonitis, wherein inhalation of gastric contents causes inflammation without the subsequent development of bacterial infection.[7, 8] Central to the study of aspiration pneumonia is whether it should exist as its own entity, or if aspiration is really a designation used for pneumonia in an older patient with greater comorbidities. The ability to clearly understand how a clinician diagnoses aspiration pneumonia, and whether that method has face validity with expert definitions may allow for improved future research, improved generalizability of current or past research, and possibly better clinical care.
Several validated mortality prediction models exist for community‐acquired pneumonia (CAP) using a variety of clinical predictors, but their performance in patients with aspiration pneumonia is less well characterized. Most studies validating pneumonia severity scoring systems excluded aspiration pneumonia from their study population.[9, 10, 11] Severity scoring systems for CAP may not accurately predict disease severity in patients with aspiration pneumonia. The CURB‐65[9] (confusion, uremia, respiratory rate, blood pressure, age 65 years) and the eCURB[12] scoring systems are poor predictors of mortality in patients with aspiration pneumonia, perhaps because they do not account for patient comorbidities.[13] The pneumonia severity index (PSI)[10] might predict mortality better than CURB‐65 in the aspiration population due to the inclusion of comorbidities.
Previous studies have demonstrated that patients with aspiration pneumonia are older and have greater disease severity and more comorbidities.[13, 14, 15] These single‐center studies also demonstrated greater mortality, more frequent admission to an intensive care unit (ICU), and longer hospital lengths of stay in patients with aspiration pneumonia. These studies identified aspiration pneumonia by the presence of a risk factor for aspiration[15] or by physician billing codes.[13] In practice, however, the bedside clinician diagnoses a patient as having aspiration pneumonia, but the logic is likely vague and inconsistent. Despite the potential for variability with individual judgment, an aggregate estimation from independent judgments may perform better than individual judgments.[16] Because there is no gold standard for defining aspiration pneumonia, all previous research has been limited to definitions created by investigators. This multicenter study seeks to determine what clinical characteristics lead physicians to diagnose a patient as having aspiration pneumonia, and whether or not the clinician‐derived diagnosis is distinct and clinically useful.
Our objectives were to: (1) identify covariates associated with bedside clinicians diagnosing a pneumonia patient as having aspiration pneumonia; (2) compare aspiration pneumonia and nonaspiration pneumonia in regard to disease severity, patient demographics, comorbidities, and clinical outcomes; and (3) measure the performance of the PSI in aspiration pneumonia versus nonaspiration pneumonia.
PATIENTS AND METHODS
Study Design and Setting
We performed a secondary analysis of the Community‐Acquired Pneumonia Organization (CAPO) database, which contains retrospectively collected data from 71 hospitals in 16 countries between June 2001 and December 2012. In each participating center, primary investigators selected nonconsecutive, adult hospitalized patients diagnosed with CAP. To decrease systematic selection biases, the selection of patients with CAP for enrollment in the trial was based on the date of hospital admission. Each investigator completed a case report form that was transferred via the internet to the CAPO study center at the University of Louisville (Louisville, KY). A sample of the data collection form is available at the study website (
Inclusion and Exclusion Criteria
Patients 18 years of age and satisfying criteria for CAP were included in this study. A diagnosis of CAP required a new pulmonary infiltrate at time of hospitalization, and at least 1 of the following: new or increased cough; leukocytosis; leukopenia, or left shift pattern on white blood cell count; and temperature >37.8C or <35.6 C. We excluded patients with pneumonia attributed to mycobacterial or fungal infection, and patients infected with human immunodeficiency virus, as we believed these types of pneumonia differ fundamentally from typical CAP.
Patient Variables
Patient variables included presence of aspiration pneumonia, laboratory data, comorbidities, and measures of disease severity, including the PSI. The clinician made a clinical diagnosis of the presence or absence of aspiration for each patient by marking a box on the case report form. Outcomes included in‐hospital mortality, hospital length of stay up to 14 days, and time to clinical stability up to 8 days. All variables were obtained directly from the case report form. In accordance with previously published definitions, we defined clinical stability as the day the following criteria were all met: improved clinical signs (improved cough and shortness of breath), lack of fever for >8 hours, improving leukocytosis (decreased at least 10% from the previous day), and tolerating oral intake.[17, 18]
Statistical Analysis
Baseline characteristics of patients with aspiration and nonaspiration CAP were compared using 2 or Fisher exact tests for categorical variables and the Mann‐Whitney U test for continuous variables.
To determine which patient variables were important in the physician diagnosis of aspiration pneumonia, we performed logistic regression with initial covariates comprising the demographic, comorbidity, and disease severity measurements listed in Table 1. We included interactions between cerebrovascular disease and age, nursing home status, and confusion to improve model fit. We centered all variables (including binary indicators) according to the method outlined by Kraemer and Blasey to improve interpretation of the main effects.[19]
Aspiration Pneumonia, N=451 | Nonaspiration Pneumonia, N=4,734 | P Value | |
---|---|---|---|
|
|||
Demographics | |||
Age, y | 79 (6587) | 69 (5380) | <0.001 |
% Male | 59% | 60% | 0.58 |
Nursing home residence | 25% | 5% | <0.001 |
Recent (30 days) antibiotic use | 21% | 16% | 0.017 |
Comorbidities | |||
Cerebrovascular disease | 35% | 14% | <0.001 |
Chronic obstructive pulmonary disease | 25% | 27% | 0.62 |
Congestive heart failure | 23% | 19% | 0.027 |
Diabetes | 18% | 18% | 0.85 |
Cancer | 12% | 10% | 0.12 |
Renal disease | 10% | 11% | 0.53 |
Liver disease | 6% | 5% | 0.29 |
Disease severity | |||
Pneumonia severity index | 123 (99153) | 92 (68117) | <0.001 |
Confusion | 49% | 12% | <0.001 |
PaO2 <60 mm Hg | 43% | 33% | <0.001 |
BUN >30 g/dL | 42% | 23% | <0.001 |
Multilobar pneumonia | 34% | 28% | 0.003 |
Pleural effusion | 25% | 21% | 0.07 |
Respiratory rate >30 breaths/minute | 21% | 20% | 0.95 |
pH <7.35 | 13% | 5% | <0.001 |
Hematocrit <30% | 11% | 6% | 0.001 |
Temperature >37.8C or <35.6C | 9% | 7% | 0.30 |
Systolic blood pressure <90 mm Hg | 8% | 9% | 0.003 |
Sodium <130 mEq/L | 8% | 6% | 0.08 |
Heart rate >125 beats/minute | 8% | 5% | 0.71 |
Glucose >250 mg/dL | 6% | 7% | 0.06 |
Cavitary lesion | 0% | 0% | 0.67 |
Clinical outcomes | |||
In‐hospital mortality | 23% | 9% | <0.001 |
Intensive care unit admission | 19% | 13% | 0.002 |
Hospital length of stay, d | 9 (515) | 7 (412) | <0.001 |
Time to clinical stability, d | 8 (48) | 4 (38) | <0.001 |
To determine if aspiration pneumonia had worse clinical outcomes compared to nonaspiration pneumonia, multiple methods were used. To compare the differences between the 2 groups with respect to time to clinical stability and length of hospital stay, we constructed Kaplan‐Meier survival curves and Cox proportional hazards regression models. The log‐rank test was used to determine statistical differences between the Kaplan‐Meier survival curves. To compare the impact of aspiration on mortality in patients with CAP, we conducted a propensity scorematched analysis. We chose propensity score matching over traditional logistic regression to balance variables among groups and to avoid the potential for overfit and multicollinearity. We considered a variable balanced after matching if its standardized difference was <10. All variables in the propensity scorematched analysis were balanced.
Although our dataset contained minimal missing data, we imputed any missing values to maintain the full study population in the creation of the propensity score. Missing data were imputed using the aregImpute function of the hmisc package of R (The R Foundation for Statistical Computing, Vienna, Austria).[20, 21] We built the propensity score model using a variable selection algorithm described by Bursac et al.[22] Our model included variables for region (United States/Canada, Europe, Asia/Africa or Latin America) and the variables listed in Table 1, with the exception of the PSI and the 4 clinical outcomes. Given that previous analyses accounting for clustering by physician did not substantially affect our results,[23] our model did not include physician‐level variables and did not account for the clustering effects of physicians. Using the propensity scores generated from this model, we matched a case of aspiration CAP with a case of nonaspiration CAP.[24] We then constructed a general linear model using the matched dataset to obtain the magnitude of effect of aspiration on mortality.
We used receiver operating characteristic curves to define the diagnostic accuracy of the pneumonia severity index for the prediction of mortality among patients with aspiration pneumonia and those with nonaspiration pneumonia. SAS version 9.3 (SAS Institute, Cary, NC) and R version 2.15.3 (The R Foundation for Statistical Computing) were used for all analyses. P values of 0.05 were considered statistically significant in all analyses.
RESULTS
Our initial query, after exclusion criteria, yielded a study population of 5185 patients (Figure 1). We compared 451 patients diagnosed with aspiration pneumonia to 4734 with CAP (Figure 1). Patient characteristics are summarized in Table 1. Patients with aspiration pneumonia were older, more likely to live in a nursing home, had greater disease severity, and were more likely to be admitted to an ICU. Patients with aspiration pneumonia had longer adjusted hospital lengths of stay and took more days to achieve clinical stability than patients with nonaspiration pneumonia (Figure 2). After adjusting for all variables in Table 1, the Cox proportional hazards models demonstrated that aspiration pneumonia was associated with ongoing hospitalization (hazard ratio [HR] for discharge: 0.77, 95% confidence interval [CI]: 0.65‐0.91, P=0.002) and clinical instability (HR for attaining clinical stability: 0.72, 95% CI: 0.61‐0.84, P<0.001). Patients with aspiration pneumonia presented with greater disease severity than those with nonaspiration pneumonia. Although there was no difference between groups in regard to temperature, respiratory rate, hyponatremia, or presence of pleural effusions or cavitary lesions, all other measured indices of disease severity were worse in patients with aspiration pneumonia. Patients with aspiration pneumonia were more likely to have cerebrovascular disease than those with nonaspiration pneumonia. Aspiration pneumonia patients also had increased prevalence of congestive heart failure. There was no appreciable difference between groups among other measured comorbidities.
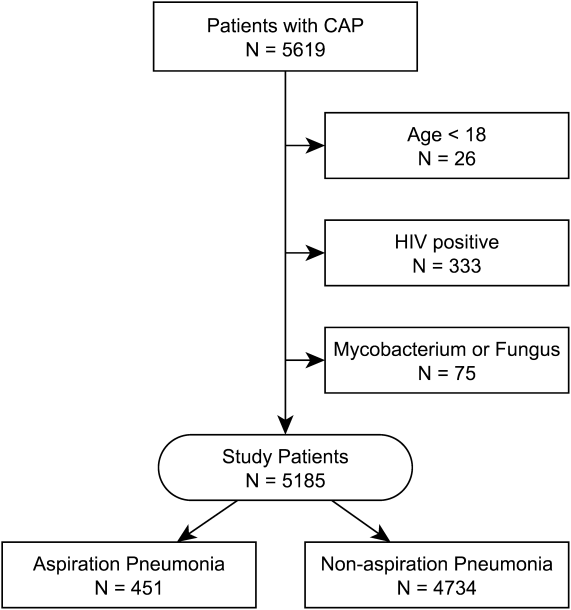
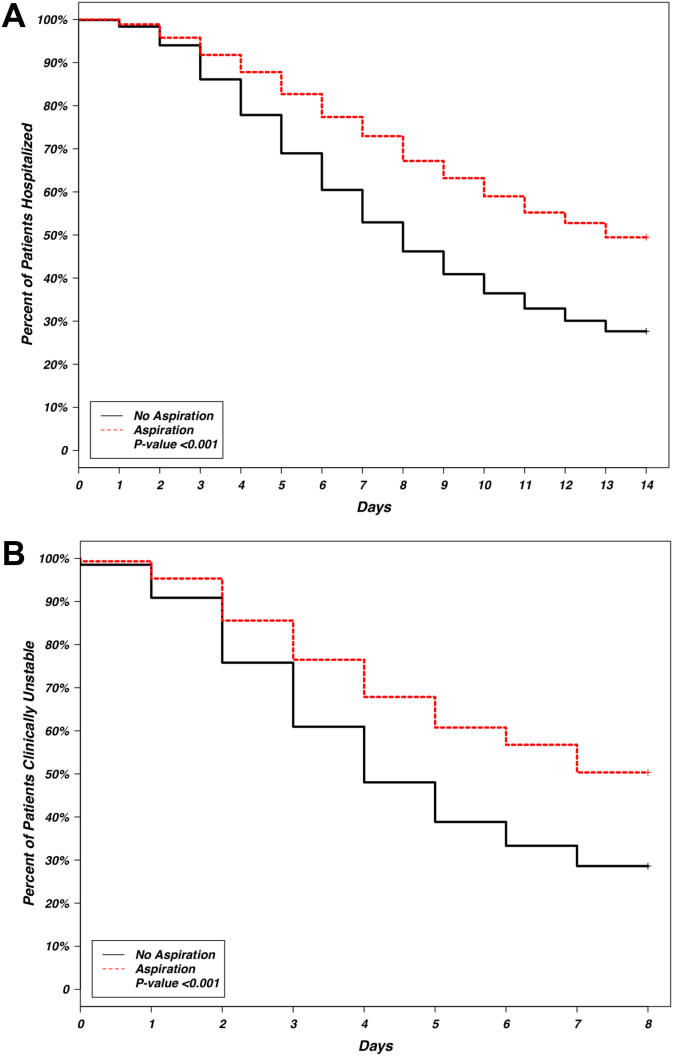
The patient characteristics most associated with a physician diagnosis of aspiration pneumonia, identified using logistic regression, were confusion, residence in nursing home, and presence of cerebrovascular disease (odds ratio [OR]: of 4.4, 2.9, and 2.3, respectively), whereas renal disease was associated with decreased physician diagnosis of aspiration pneumonia over nonaspiration pneumonia (OR: 0.58) (Table 2).
Covariate | Odds Ratio | 95% Confidence Intervals | P Value |
---|---|---|---|
|
|||
Demographics | |||
Age, y | 1.00 | 0.991.01 | 0.948 |
Male | 1.20 | 0.941.54 | 0.148 |
Nursing home residence | 2.93 | 2.134.00 | <0.001 |
Comorbidities | |||
Cerebrovascular disease | 2.26 | 1.533.32 | <0.001 |
Renal disease | 0.58 | 0.390.85 | 0.006 |
Disease severity | |||
Confusion | 4.41 | 3.405.72 | <0.001 |
Hematocrit <30% | 1.59 | 1.062.33 | 0.020 |
pH <7.35 | 1.67 | 1.102.47 | 0.013 |
Temperature >37.8C or <35.6C | 1.60 | 1.072.35 | 0.019 |
Multilobar pneumonia | 1.29 | 1.001.65 | 0.047 |
Interaction terms | |||
Age * cerebrovascular disease | 0.98 | 0.960.99 | 0.011 |
Nursing home * cerebrovascular disease | 0.51 | 0.270.96 | 0.037 |
Confusion * cerebrovascular disease | 0.70 | 0.421.17 | 0.175 |
Observed in‐patient mortality of aspiration pneumonia was 23%. This mortality was considerably higher than a mean PSI score of 123 would predict (class IV risk group, with expected 30‐day mortality of 8%9%[25]). The PSI score's ability to predict inpatient mortality in patients with aspiration pneumonia was moderate, with an area under the curve (AUC) of 0.71. This was similar to its performance in patients with nonaspiration pneumonia (AUC of 0.75) (Figure 3). These values are lower than the AUC of 0.81 for the PSI in predicting mortality derived from a meta‐analysis of 31 other studies.[26]
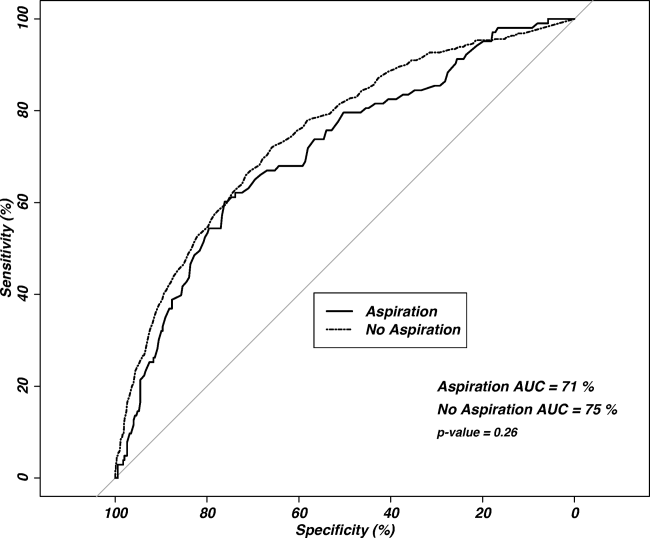
Our regression model after propensity score matching demonstrated that aspiration pneumonia independently confers a 2.3‐fold increased odds for inpatient mortality (95% CI: 1.56‐3.45, P<0.001).
DISCUSSION
Pneumonia patients with confusion, nursing home residence, or cerebrovascular disease are more likely to be diagnosed with aspiration pneumonia by clinicians. Although this is unsurprising, it is notable that these patients are more than twice as likely to die in the inpatient setting, even after accounting for age, comorbidities, and disease severity. These findings are similar to three previously published studies comparing aspiration and nonaspiration pneumonia at single institutions, albeit using different aspiration pneumonia definitions.[13, 14, 15] This study is the first large, multicenter, multinational study to demonstrate these findings.
Central to the interpretation of our results is the method of diagnosing aspiration versus nonaspiration. A bottom‐up method that relies on a clinician to check a box for aspiration may appear poorly reproducible. Because there is no diagnostic gold standard, clinicians may use different criteria to diagnose aspiration, creating potential for idiosyncratic noise. The strength of the wisdom of the crowd method used in this study is that an aggregate estimation from independent judgments may reduce the noise from individual judgments.[16] Although clinicians may vary in why they diagnose a particular patient as having aspiration pneumonia, it appears that the overwhelming reason for diagnosing a patient as having aspiration pneumonia is the presence of confusion, followed by previous nursing home residence or cerebrovascular disease. This finding has some face validity when compared with studies using an investigator definition, as altered mental status, chronic debility, and cerebrovascular disease are either prominent features of the definition of aspiration pneumonia[8] or frequently observed in patients with aspiration pneumonia.[13, 15] The distribution of cerebrovascular disease among our study's aspiration and nonaspiration pneumonia patients was similar to studies that used formal criteria in their definitions.[13, 15] Although nursing home residence was more likely in aspiration pneumonia patients, the majority of aspiration pneumonia patients were residing in the community, suggesting that aspiration is not simply a surrogate for healthcare‐associated pneumonia. Although patients with aspiration pneumonia are typically older than their nonaspiration counterparts, it appears that age is not a key determinant in the diagnosis of aspiration. With aspiration pneumonia, confusion, nursing home residence, and the presence of cerebrovascular disease are the greatest contributors in the clinical diagnosis, more than age.
Our data demonstrate that aspiration pneumonia confers increased odds for mortality, even after adjustment for age, disease severity, and comorbidities. These data suggest that aspiration pneumonia is a distinct entity from nonaspiration pneumonia, and that this disease is worse than nonaspiration CAP. If aspiration pneumonia is distinct from nonaspiration pneumonia, some unrecognized host factor other than age, disease severity, or the captured comorbidities decreases survival in aspiration pneumonia patients. However, it is also possible that aspiration pneumonia is merely a clinical designation for one end of the pneumonia spectrum, and we and others have failed to completely account for all measures of disease severity or all measures of comorbidities. Examples of unmeasured comorbidities would include presence of oropharyngeal dysphagia, which is not assessed in the database but could have a significant effect on clinical diagnosis. Unmeasured covariates can include measures beyond that of disease severity or comorbidity, such as the presence of a do not resuscitate (DNR) order, which could have a significant confounding effect on the observed association. A previous, single‐center study demonstrated that increased 30‐day mortality in aspiration pneumonia was mostly attributable to greater disease severity and comorbidities, although aspiration pneumonia independently conferred greater risk for adverse long‐term outcomes.[15] We propose that aspiration pneumonia represents a clinically distinct entity from nonaspiration pneumonia. Patients with chronic aspiration are often chronically malnourished and may have different oral flora than patients without chronic aspiration.[27, 28] Chronic aspiration has been associated with granulomatous reaction, organizing pneumonia, diffuse alveolar damage, and chronic bronchiolitis.[29] Chronic aspiration may elicit changes in the host physiology, and may render the host more susceptible to the development of secondary bacterial infection with morbid consequences.
The ability of the PSI to predict inpatient mortality was moderate (AUC only 0.7), with no significant additional discrimination between the aspiration and nonaspiration pneumonia groups. Although the PSI had moderate ability to predict inpatient mortality, the observed mortality was considerably higher than predicted. It is possible that the PSI incompletely captures clinically relevant comorbidities (eg, malnutrition). Further study to improve mortality prediction of aspiration pneumonia patients could employ sensitivity analysis to determine optimal thresholds and weighting of the PSI components.
Patients with aspiration pneumonia had longer hospital lengths of stay and took longer to achieve clinical stability than their nonaspiration counterparts. Time to clinical stability has been associated with increased posthospitalization mortality and is associated with time to switch from intravenous to oral antibiotics.[17] Although some component of hospital length‐of‐stay is subject to local practice patterns, time to clinical stability has explicit criteria for clinical improvement and failure, and therefore is less likely to be affected by local practice patterns.
We noted a relatively high (16%21%) incidence of prior antibiotic use among patients in this database. Analysis of antibiotic prescription patterns was limited, given the several different countries from which the database draws its cases. Although we used accepted criteria to define CAP cases, it is possible that this population may have a higher rate of resistant or uncommon pathogens than other studies of CAP that have populations with lower incidence of prior antibiotic use. Although not assessed, we suspect a significant component of the prior antibiotic use represented outpatient pneumonia treatment during the few days prior to visiting the hospital.
This study has several limitations, of which the most important may be that we used clinical determination for defining presence of aspiration pneumonia. This method is susceptible to the subjective perceptions of the treating clinician. We did not account for the effect of individual physicians in our model, although we did adjust for regional differences. The retrospective identification of patients allows for the possibility of selection bias, and therefore we have not attempted to make inferences regarding the relative incidence of pneumonia, nor did we adjust for temporal trends in diagnosis. The ratio of aspiration pneumonia patients to nonaspiration pneumonia patients may not necessarily reflect that observed in reality. Microbiologic and antibiotic data were unavailable for analysis. This study cannot inform on nonhospitalized patients with aspiration pneumonia, as only hospitalized patients were enrolled. The database identified cases of pneumonia, so it is possible for a patient to enter into the database more than once. Detection of mortality was limited to the inpatient setting rather than a set interval of 30 days. Inpatient mortality depends on length‐of‐stay patterns that may bias the mortality endpoint.[30] Also not assessed was the presence of a DNR order. It is possible that an older patient with greater comorbidities and disease severity may have care intentionally limited or withdrawn early by the family or clinicians.
Strengths of the study include its size and its multicenter, multinational population. The CAPO database is a large and well‐described population of patients with CAP.[17, 31] These attributes, as well as the clinician‐determined diagnosis, increase the generalizability of the study compared to a single‐center, single‐country study that employs investigator‐defined criteria.
CONCLUSION
Pneumonia patients with confusion, who are nursing home residence, and have cerebrovascular disease are more likely to be diagnosed with aspiration pneumonia by clinicians. Our clinician‐diagnosed cohort appears similar to those derived using an investigator definition. Patients with aspiration pneumonia are older, and have greater disease severity and more comorbidities than patients with nonaspiration pneumonia. They have greater mortality than their PSI score class would predict. Even after accounting for age, disease severity, and comorbidities, the presence of aspiration pneumonia independently conferred a greater than 2‐fold increase in inpatient mortality. These findings together suggest that aspiration pneumonia should be considered a distinct entity from typical pneumonia, and that additional research should be done in this field.
ACKNOWLEDGMENTS
Disclosures: M.J.L. contributed to the study design, data analysis, statistical analysis, and writing of the manuscript. P.P. contributed to the study design and revision of the manuscript for important intellectual content. T.W. and E.W. contributed to the study design, statistical analysis, and revision of the manuscript for important intellectual content. J.A.R. and N.C.D. contributed to the study design and revision of the manuscript for important intellectual content. All authors read and approved the final manuscript. M.L. takes responsibility for the integrity of the work as a whole, from inception to published article. This investigation was partly supported with funding from the National Center for Research Resources and the National Center for Advancing Translational Sciences, National Institutes of Health (grant 8UL1TR000105 [formerly UL1RR025764]). The authors report no conflicts of interest.
- Severe community‐acquired pneumonia. Epidemiology and prognostic factors. Am Rev Respir Dis. 1991;144(2):312–318. , , , et al.
- Risk factors for pneumonia in the elderly. Am J Med. 1994;96(4):313–320. , , .
- Aspiration pneumonia and dysphagia in the elderly. Chest. 2003;124(1):328–336. , .
- Pneumonia versus aspiration pneumonitis in nursing home residents: diagnosis and management. J Am Geriatr Soc. 2003;51(1):17–23. , , .
- Aspiration pneumonia: mixing apples with oranges and tangerines. Crit Care Med. 2004;32(5):1236; author reply 1236–1237. .
- Epidemiology and impact of aspiration pneumonia in patients undergoing surgery in Maryland, 1999–2000. Crit Care Med. 2003;31(7):1930–1937. , , , , .
- Aspiration syndromes: aspiration pneumonia and pneumonitis. Hosp Pract (Minneap). 2010;38(1):35–42. .
- Aspiration pneumonitis and aspiration pneumonia. N Engl J Med. 2001;344(9):665–671. .
- Defining community acquired pneumonia severity on presentation to hospital: an international derivation and validation study. Thorax. 2003;58(5):377–382. , , , et al.
- Comparison of a disease‐specific and a generic severity of illness measure for patients with community‐acquired pneumonia. J Gen Intern Med. 1995;10(7):359–368. , , , et al.
- Development and validation of a clinical prediction rule for severe community‐acquired pneumonia. Am J Respir Crit Care Med. 2006;174(11):1249–1256. , , , et al.
- CURB‐65 pneumonia severity assessment adapted for electronic decision support. Chest. 2011;140(1):156–163. , , , et al.
- Mortality, morbidity, and disease severity of patients with aspiration pneumonia. J Hosp Med. 2013;8(2):83–90. , , , .
- Pneumonia Severity Index (PSI), CURB‐65, and mortality in hospitalized elderly patients with aspiration pneumonia [in German]. Z Gerontol Geriatr. 2011;44(4):229–234. , , , , .
- Risk factors for aspiration in community‐acquired pneumonia: analysis of a hospitalized UK cohort. Am J Med. 2013;126(11):995–1001. , , , , .
- The wisdom of the crowd in combinatorial problems. Cogn Sci. 2012;36(3):452–470. , , , .
- Association between time to clinical stability and outcomes after discharge in hospitalized patients with community‐acquired pneumonia. Chest. 2011;140(2):482–488. , , , et al.
- Clinical stability and switch therapy in hospitalised patients with community‐acquired pneumonia: are we there yet? Eur Respir J. 2013;41(1):5–6. .
- Centring in regression analyses: a strategy to prevent errors in statistical inference. Int J Methods Psychiatr Res. 2004;13(3):141–151. , .
- http://CRAN.R‐project.org/package=Hmisc. Published Sept 12, 2014. Last accessed Oct 27, 2014. . Hmisc: Harrell miscellaneous. Available at:
- Multiple imputation for the fatal accident reporting system. J R Stat Soc Ser C Appl Stat. 1991;40(1):13–29. , .
- Purposeful selection of variables in logistic regression. Source Code Biol Med. 2008;3:17. , , , .
- CAPO authors. Mortality differences among hospitalized patients with community‐acquired pneumonia in three world regions: results from the Community‐Acquired Pneumonia Organization (CAPO) International Cohort Study. Respir Med. 2013;107(7):1101–1111. , , , , ;
- 2001:214–226. Available at: http://www2.sas.com/proceedings/sugi26/p214–26.pdf. Last accessed Oct 27, 2014. . Reducing bias in a propensity score matched‐pair sample using greedy matching techniques. In: Proceedings of the 26th Annual SAS Users Group International Conference. Cary, NC: SAS Institute Inc.;
- A prediction rule to identify low‐risk patients with community‐acquired pneumonia. N Engl J Med. 1997;336(4):243–250. , , , et al.
- Severity assessment tools for predicting mortality in hospitalised patients with community‐acquired pneumonia. Systematic review and meta‐analysis. Thorax. 2010;65(10):878–883. , , , et al.
- Prevalence and prognostic implications of dysphagia in elderly patients with pneumonia. Age Ageing. 2010;39(1):39–45. , , , , , .
- The association between oral microorgansims and aspiration pneumonia in the institutionalized elderly: review and recommendations. Dysphagia. 2010;25(4):307–322. , .
- Histopathology of aspiration pneumonia not associated with food or other particulate matter: a clinicopathologic study of 10 cases diagnosed on biopsy. Am J Surg Pathol. 2011;35(3):426–431. , .
- Interpreting hospital mortality data. The role of clinical risk adjustment. JAMA. 1988;260(24):3611–3616. , , , , , .
- Hospitalization for community‐acquired pneumonia: the pneumonia severity index vs clinical judgment. Chest. 2003;124(1):121–124. , , , .
Pneumonia is a common clinical syndrome with well‐described epidemiology and microbiology. Aspiration pneumonia comprises 5% to 15% of patients with pneumonia acquired outside of the hospital,[1] but is less well characterized despite being a major syndrome of pneumonia in the elderly.[2, 3] Difficulties in studying aspiration pneumonia include the lack of a sensitive and specific marker for aspiration as well as the potential overlap between aspiration pneumonia and other forms of pneumonia.[4, 5, 6] Additionally, clinicians have difficulty distinguishing between aspiration pneumonia, which develops after the aspiration of oropharyngeal contents, and aspiration pneumonitis, wherein inhalation of gastric contents causes inflammation without the subsequent development of bacterial infection.[7, 8] Central to the study of aspiration pneumonia is whether it should exist as its own entity, or if aspiration is really a designation used for pneumonia in an older patient with greater comorbidities. The ability to clearly understand how a clinician diagnoses aspiration pneumonia, and whether that method has face validity with expert definitions may allow for improved future research, improved generalizability of current or past research, and possibly better clinical care.
Several validated mortality prediction models exist for community‐acquired pneumonia (CAP) using a variety of clinical predictors, but their performance in patients with aspiration pneumonia is less well characterized. Most studies validating pneumonia severity scoring systems excluded aspiration pneumonia from their study population.[9, 10, 11] Severity scoring systems for CAP may not accurately predict disease severity in patients with aspiration pneumonia. The CURB‐65[9] (confusion, uremia, respiratory rate, blood pressure, age 65 years) and the eCURB[12] scoring systems are poor predictors of mortality in patients with aspiration pneumonia, perhaps because they do not account for patient comorbidities.[13] The pneumonia severity index (PSI)[10] might predict mortality better than CURB‐65 in the aspiration population due to the inclusion of comorbidities.
Previous studies have demonstrated that patients with aspiration pneumonia are older and have greater disease severity and more comorbidities.[13, 14, 15] These single‐center studies also demonstrated greater mortality, more frequent admission to an intensive care unit (ICU), and longer hospital lengths of stay in patients with aspiration pneumonia. These studies identified aspiration pneumonia by the presence of a risk factor for aspiration[15] or by physician billing codes.[13] In practice, however, the bedside clinician diagnoses a patient as having aspiration pneumonia, but the logic is likely vague and inconsistent. Despite the potential for variability with individual judgment, an aggregate estimation from independent judgments may perform better than individual judgments.[16] Because there is no gold standard for defining aspiration pneumonia, all previous research has been limited to definitions created by investigators. This multicenter study seeks to determine what clinical characteristics lead physicians to diagnose a patient as having aspiration pneumonia, and whether or not the clinician‐derived diagnosis is distinct and clinically useful.
Our objectives were to: (1) identify covariates associated with bedside clinicians diagnosing a pneumonia patient as having aspiration pneumonia; (2) compare aspiration pneumonia and nonaspiration pneumonia in regard to disease severity, patient demographics, comorbidities, and clinical outcomes; and (3) measure the performance of the PSI in aspiration pneumonia versus nonaspiration pneumonia.
PATIENTS AND METHODS
Study Design and Setting
We performed a secondary analysis of the Community‐Acquired Pneumonia Organization (CAPO) database, which contains retrospectively collected data from 71 hospitals in 16 countries between June 2001 and December 2012. In each participating center, primary investigators selected nonconsecutive, adult hospitalized patients diagnosed with CAP. To decrease systematic selection biases, the selection of patients with CAP for enrollment in the trial was based on the date of hospital admission. Each investigator completed a case report form that was transferred via the internet to the CAPO study center at the University of Louisville (Louisville, KY). A sample of the data collection form is available at the study website (
Inclusion and Exclusion Criteria
Patients 18 years of age and satisfying criteria for CAP were included in this study. A diagnosis of CAP required a new pulmonary infiltrate at time of hospitalization, and at least 1 of the following: new or increased cough; leukocytosis; leukopenia, or left shift pattern on white blood cell count; and temperature >37.8C or <35.6 C. We excluded patients with pneumonia attributed to mycobacterial or fungal infection, and patients infected with human immunodeficiency virus, as we believed these types of pneumonia differ fundamentally from typical CAP.
Patient Variables
Patient variables included presence of aspiration pneumonia, laboratory data, comorbidities, and measures of disease severity, including the PSI. The clinician made a clinical diagnosis of the presence or absence of aspiration for each patient by marking a box on the case report form. Outcomes included in‐hospital mortality, hospital length of stay up to 14 days, and time to clinical stability up to 8 days. All variables were obtained directly from the case report form. In accordance with previously published definitions, we defined clinical stability as the day the following criteria were all met: improved clinical signs (improved cough and shortness of breath), lack of fever for >8 hours, improving leukocytosis (decreased at least 10% from the previous day), and tolerating oral intake.[17, 18]
Statistical Analysis
Baseline characteristics of patients with aspiration and nonaspiration CAP were compared using 2 or Fisher exact tests for categorical variables and the Mann‐Whitney U test for continuous variables.
To determine which patient variables were important in the physician diagnosis of aspiration pneumonia, we performed logistic regression with initial covariates comprising the demographic, comorbidity, and disease severity measurements listed in Table 1. We included interactions between cerebrovascular disease and age, nursing home status, and confusion to improve model fit. We centered all variables (including binary indicators) according to the method outlined by Kraemer and Blasey to improve interpretation of the main effects.[19]
Aspiration Pneumonia, N=451 | Nonaspiration Pneumonia, N=4,734 | P Value | |
---|---|---|---|
|
|||
Demographics | |||
Age, y | 79 (6587) | 69 (5380) | <0.001 |
% Male | 59% | 60% | 0.58 |
Nursing home residence | 25% | 5% | <0.001 |
Recent (30 days) antibiotic use | 21% | 16% | 0.017 |
Comorbidities | |||
Cerebrovascular disease | 35% | 14% | <0.001 |
Chronic obstructive pulmonary disease | 25% | 27% | 0.62 |
Congestive heart failure | 23% | 19% | 0.027 |
Diabetes | 18% | 18% | 0.85 |
Cancer | 12% | 10% | 0.12 |
Renal disease | 10% | 11% | 0.53 |
Liver disease | 6% | 5% | 0.29 |
Disease severity | |||
Pneumonia severity index | 123 (99153) | 92 (68117) | <0.001 |
Confusion | 49% | 12% | <0.001 |
PaO2 <60 mm Hg | 43% | 33% | <0.001 |
BUN >30 g/dL | 42% | 23% | <0.001 |
Multilobar pneumonia | 34% | 28% | 0.003 |
Pleural effusion | 25% | 21% | 0.07 |
Respiratory rate >30 breaths/minute | 21% | 20% | 0.95 |
pH <7.35 | 13% | 5% | <0.001 |
Hematocrit <30% | 11% | 6% | 0.001 |
Temperature >37.8C or <35.6C | 9% | 7% | 0.30 |
Systolic blood pressure <90 mm Hg | 8% | 9% | 0.003 |
Sodium <130 mEq/L | 8% | 6% | 0.08 |
Heart rate >125 beats/minute | 8% | 5% | 0.71 |
Glucose >250 mg/dL | 6% | 7% | 0.06 |
Cavitary lesion | 0% | 0% | 0.67 |
Clinical outcomes | |||
In‐hospital mortality | 23% | 9% | <0.001 |
Intensive care unit admission | 19% | 13% | 0.002 |
Hospital length of stay, d | 9 (515) | 7 (412) | <0.001 |
Time to clinical stability, d | 8 (48) | 4 (38) | <0.001 |
To determine if aspiration pneumonia had worse clinical outcomes compared to nonaspiration pneumonia, multiple methods were used. To compare the differences between the 2 groups with respect to time to clinical stability and length of hospital stay, we constructed Kaplan‐Meier survival curves and Cox proportional hazards regression models. The log‐rank test was used to determine statistical differences between the Kaplan‐Meier survival curves. To compare the impact of aspiration on mortality in patients with CAP, we conducted a propensity scorematched analysis. We chose propensity score matching over traditional logistic regression to balance variables among groups and to avoid the potential for overfit and multicollinearity. We considered a variable balanced after matching if its standardized difference was <10. All variables in the propensity scorematched analysis were balanced.
Although our dataset contained minimal missing data, we imputed any missing values to maintain the full study population in the creation of the propensity score. Missing data were imputed using the aregImpute function of the hmisc package of R (The R Foundation for Statistical Computing, Vienna, Austria).[20, 21] We built the propensity score model using a variable selection algorithm described by Bursac et al.[22] Our model included variables for region (United States/Canada, Europe, Asia/Africa or Latin America) and the variables listed in Table 1, with the exception of the PSI and the 4 clinical outcomes. Given that previous analyses accounting for clustering by physician did not substantially affect our results,[23] our model did not include physician‐level variables and did not account for the clustering effects of physicians. Using the propensity scores generated from this model, we matched a case of aspiration CAP with a case of nonaspiration CAP.[24] We then constructed a general linear model using the matched dataset to obtain the magnitude of effect of aspiration on mortality.
We used receiver operating characteristic curves to define the diagnostic accuracy of the pneumonia severity index for the prediction of mortality among patients with aspiration pneumonia and those with nonaspiration pneumonia. SAS version 9.3 (SAS Institute, Cary, NC) and R version 2.15.3 (The R Foundation for Statistical Computing) were used for all analyses. P values of 0.05 were considered statistically significant in all analyses.
RESULTS
Our initial query, after exclusion criteria, yielded a study population of 5185 patients (Figure 1). We compared 451 patients diagnosed with aspiration pneumonia to 4734 with CAP (Figure 1). Patient characteristics are summarized in Table 1. Patients with aspiration pneumonia were older, more likely to live in a nursing home, had greater disease severity, and were more likely to be admitted to an ICU. Patients with aspiration pneumonia had longer adjusted hospital lengths of stay and took more days to achieve clinical stability than patients with nonaspiration pneumonia (Figure 2). After adjusting for all variables in Table 1, the Cox proportional hazards models demonstrated that aspiration pneumonia was associated with ongoing hospitalization (hazard ratio [HR] for discharge: 0.77, 95% confidence interval [CI]: 0.65‐0.91, P=0.002) and clinical instability (HR for attaining clinical stability: 0.72, 95% CI: 0.61‐0.84, P<0.001). Patients with aspiration pneumonia presented with greater disease severity than those with nonaspiration pneumonia. Although there was no difference between groups in regard to temperature, respiratory rate, hyponatremia, or presence of pleural effusions or cavitary lesions, all other measured indices of disease severity were worse in patients with aspiration pneumonia. Patients with aspiration pneumonia were more likely to have cerebrovascular disease than those with nonaspiration pneumonia. Aspiration pneumonia patients also had increased prevalence of congestive heart failure. There was no appreciable difference between groups among other measured comorbidities.
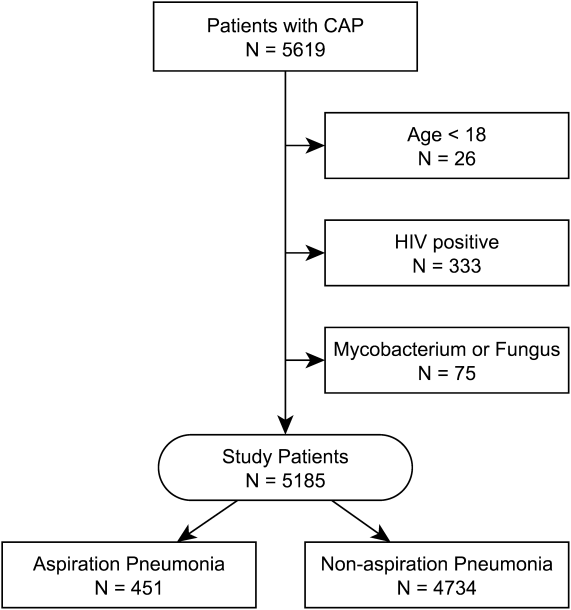
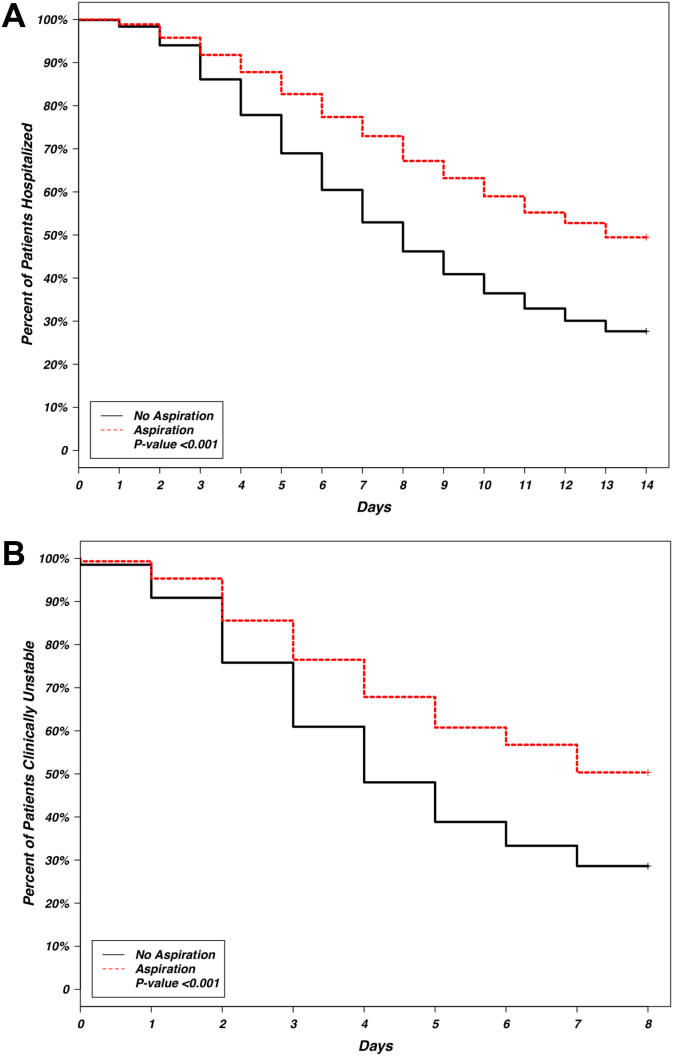
The patient characteristics most associated with a physician diagnosis of aspiration pneumonia, identified using logistic regression, were confusion, residence in nursing home, and presence of cerebrovascular disease (odds ratio [OR]: of 4.4, 2.9, and 2.3, respectively), whereas renal disease was associated with decreased physician diagnosis of aspiration pneumonia over nonaspiration pneumonia (OR: 0.58) (Table 2).
Covariate | Odds Ratio | 95% Confidence Intervals | P Value |
---|---|---|---|
|
|||
Demographics | |||
Age, y | 1.00 | 0.991.01 | 0.948 |
Male | 1.20 | 0.941.54 | 0.148 |
Nursing home residence | 2.93 | 2.134.00 | <0.001 |
Comorbidities | |||
Cerebrovascular disease | 2.26 | 1.533.32 | <0.001 |
Renal disease | 0.58 | 0.390.85 | 0.006 |
Disease severity | |||
Confusion | 4.41 | 3.405.72 | <0.001 |
Hematocrit <30% | 1.59 | 1.062.33 | 0.020 |
pH <7.35 | 1.67 | 1.102.47 | 0.013 |
Temperature >37.8C or <35.6C | 1.60 | 1.072.35 | 0.019 |
Multilobar pneumonia | 1.29 | 1.001.65 | 0.047 |
Interaction terms | |||
Age * cerebrovascular disease | 0.98 | 0.960.99 | 0.011 |
Nursing home * cerebrovascular disease | 0.51 | 0.270.96 | 0.037 |
Confusion * cerebrovascular disease | 0.70 | 0.421.17 | 0.175 |
Observed in‐patient mortality of aspiration pneumonia was 23%. This mortality was considerably higher than a mean PSI score of 123 would predict (class IV risk group, with expected 30‐day mortality of 8%9%[25]). The PSI score's ability to predict inpatient mortality in patients with aspiration pneumonia was moderate, with an area under the curve (AUC) of 0.71. This was similar to its performance in patients with nonaspiration pneumonia (AUC of 0.75) (Figure 3). These values are lower than the AUC of 0.81 for the PSI in predicting mortality derived from a meta‐analysis of 31 other studies.[26]
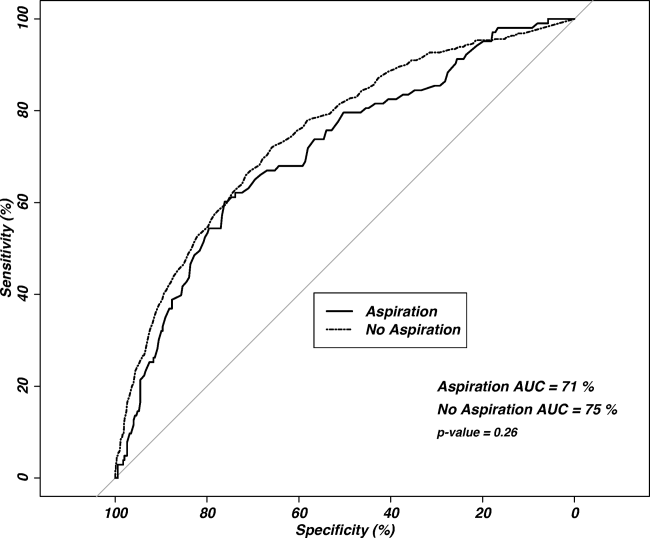
Our regression model after propensity score matching demonstrated that aspiration pneumonia independently confers a 2.3‐fold increased odds for inpatient mortality (95% CI: 1.56‐3.45, P<0.001).
DISCUSSION
Pneumonia patients with confusion, nursing home residence, or cerebrovascular disease are more likely to be diagnosed with aspiration pneumonia by clinicians. Although this is unsurprising, it is notable that these patients are more than twice as likely to die in the inpatient setting, even after accounting for age, comorbidities, and disease severity. These findings are similar to three previously published studies comparing aspiration and nonaspiration pneumonia at single institutions, albeit using different aspiration pneumonia definitions.[13, 14, 15] This study is the first large, multicenter, multinational study to demonstrate these findings.
Central to the interpretation of our results is the method of diagnosing aspiration versus nonaspiration. A bottom‐up method that relies on a clinician to check a box for aspiration may appear poorly reproducible. Because there is no diagnostic gold standard, clinicians may use different criteria to diagnose aspiration, creating potential for idiosyncratic noise. The strength of the wisdom of the crowd method used in this study is that an aggregate estimation from independent judgments may reduce the noise from individual judgments.[16] Although clinicians may vary in why they diagnose a particular patient as having aspiration pneumonia, it appears that the overwhelming reason for diagnosing a patient as having aspiration pneumonia is the presence of confusion, followed by previous nursing home residence or cerebrovascular disease. This finding has some face validity when compared with studies using an investigator definition, as altered mental status, chronic debility, and cerebrovascular disease are either prominent features of the definition of aspiration pneumonia[8] or frequently observed in patients with aspiration pneumonia.[13, 15] The distribution of cerebrovascular disease among our study's aspiration and nonaspiration pneumonia patients was similar to studies that used formal criteria in their definitions.[13, 15] Although nursing home residence was more likely in aspiration pneumonia patients, the majority of aspiration pneumonia patients were residing in the community, suggesting that aspiration is not simply a surrogate for healthcare‐associated pneumonia. Although patients with aspiration pneumonia are typically older than their nonaspiration counterparts, it appears that age is not a key determinant in the diagnosis of aspiration. With aspiration pneumonia, confusion, nursing home residence, and the presence of cerebrovascular disease are the greatest contributors in the clinical diagnosis, more than age.
Our data demonstrate that aspiration pneumonia confers increased odds for mortality, even after adjustment for age, disease severity, and comorbidities. These data suggest that aspiration pneumonia is a distinct entity from nonaspiration pneumonia, and that this disease is worse than nonaspiration CAP. If aspiration pneumonia is distinct from nonaspiration pneumonia, some unrecognized host factor other than age, disease severity, or the captured comorbidities decreases survival in aspiration pneumonia patients. However, it is also possible that aspiration pneumonia is merely a clinical designation for one end of the pneumonia spectrum, and we and others have failed to completely account for all measures of disease severity or all measures of comorbidities. Examples of unmeasured comorbidities would include presence of oropharyngeal dysphagia, which is not assessed in the database but could have a significant effect on clinical diagnosis. Unmeasured covariates can include measures beyond that of disease severity or comorbidity, such as the presence of a do not resuscitate (DNR) order, which could have a significant confounding effect on the observed association. A previous, single‐center study demonstrated that increased 30‐day mortality in aspiration pneumonia was mostly attributable to greater disease severity and comorbidities, although aspiration pneumonia independently conferred greater risk for adverse long‐term outcomes.[15] We propose that aspiration pneumonia represents a clinically distinct entity from nonaspiration pneumonia. Patients with chronic aspiration are often chronically malnourished and may have different oral flora than patients without chronic aspiration.[27, 28] Chronic aspiration has been associated with granulomatous reaction, organizing pneumonia, diffuse alveolar damage, and chronic bronchiolitis.[29] Chronic aspiration may elicit changes in the host physiology, and may render the host more susceptible to the development of secondary bacterial infection with morbid consequences.
The ability of the PSI to predict inpatient mortality was moderate (AUC only 0.7), with no significant additional discrimination between the aspiration and nonaspiration pneumonia groups. Although the PSI had moderate ability to predict inpatient mortality, the observed mortality was considerably higher than predicted. It is possible that the PSI incompletely captures clinically relevant comorbidities (eg, malnutrition). Further study to improve mortality prediction of aspiration pneumonia patients could employ sensitivity analysis to determine optimal thresholds and weighting of the PSI components.
Patients with aspiration pneumonia had longer hospital lengths of stay and took longer to achieve clinical stability than their nonaspiration counterparts. Time to clinical stability has been associated with increased posthospitalization mortality and is associated with time to switch from intravenous to oral antibiotics.[17] Although some component of hospital length‐of‐stay is subject to local practice patterns, time to clinical stability has explicit criteria for clinical improvement and failure, and therefore is less likely to be affected by local practice patterns.
We noted a relatively high (16%21%) incidence of prior antibiotic use among patients in this database. Analysis of antibiotic prescription patterns was limited, given the several different countries from which the database draws its cases. Although we used accepted criteria to define CAP cases, it is possible that this population may have a higher rate of resistant or uncommon pathogens than other studies of CAP that have populations with lower incidence of prior antibiotic use. Although not assessed, we suspect a significant component of the prior antibiotic use represented outpatient pneumonia treatment during the few days prior to visiting the hospital.
This study has several limitations, of which the most important may be that we used clinical determination for defining presence of aspiration pneumonia. This method is susceptible to the subjective perceptions of the treating clinician. We did not account for the effect of individual physicians in our model, although we did adjust for regional differences. The retrospective identification of patients allows for the possibility of selection bias, and therefore we have not attempted to make inferences regarding the relative incidence of pneumonia, nor did we adjust for temporal trends in diagnosis. The ratio of aspiration pneumonia patients to nonaspiration pneumonia patients may not necessarily reflect that observed in reality. Microbiologic and antibiotic data were unavailable for analysis. This study cannot inform on nonhospitalized patients with aspiration pneumonia, as only hospitalized patients were enrolled. The database identified cases of pneumonia, so it is possible for a patient to enter into the database more than once. Detection of mortality was limited to the inpatient setting rather than a set interval of 30 days. Inpatient mortality depends on length‐of‐stay patterns that may bias the mortality endpoint.[30] Also not assessed was the presence of a DNR order. It is possible that an older patient with greater comorbidities and disease severity may have care intentionally limited or withdrawn early by the family or clinicians.
Strengths of the study include its size and its multicenter, multinational population. The CAPO database is a large and well‐described population of patients with CAP.[17, 31] These attributes, as well as the clinician‐determined diagnosis, increase the generalizability of the study compared to a single‐center, single‐country study that employs investigator‐defined criteria.
CONCLUSION
Pneumonia patients with confusion, who are nursing home residence, and have cerebrovascular disease are more likely to be diagnosed with aspiration pneumonia by clinicians. Our clinician‐diagnosed cohort appears similar to those derived using an investigator definition. Patients with aspiration pneumonia are older, and have greater disease severity and more comorbidities than patients with nonaspiration pneumonia. They have greater mortality than their PSI score class would predict. Even after accounting for age, disease severity, and comorbidities, the presence of aspiration pneumonia independently conferred a greater than 2‐fold increase in inpatient mortality. These findings together suggest that aspiration pneumonia should be considered a distinct entity from typical pneumonia, and that additional research should be done in this field.
ACKNOWLEDGMENTS
Disclosures: M.J.L. contributed to the study design, data analysis, statistical analysis, and writing of the manuscript. P.P. contributed to the study design and revision of the manuscript for important intellectual content. T.W. and E.W. contributed to the study design, statistical analysis, and revision of the manuscript for important intellectual content. J.A.R. and N.C.D. contributed to the study design and revision of the manuscript for important intellectual content. All authors read and approved the final manuscript. M.L. takes responsibility for the integrity of the work as a whole, from inception to published article. This investigation was partly supported with funding from the National Center for Research Resources and the National Center for Advancing Translational Sciences, National Institutes of Health (grant 8UL1TR000105 [formerly UL1RR025764]). The authors report no conflicts of interest.
Pneumonia is a common clinical syndrome with well‐described epidemiology and microbiology. Aspiration pneumonia comprises 5% to 15% of patients with pneumonia acquired outside of the hospital,[1] but is less well characterized despite being a major syndrome of pneumonia in the elderly.[2, 3] Difficulties in studying aspiration pneumonia include the lack of a sensitive and specific marker for aspiration as well as the potential overlap between aspiration pneumonia and other forms of pneumonia.[4, 5, 6] Additionally, clinicians have difficulty distinguishing between aspiration pneumonia, which develops after the aspiration of oropharyngeal contents, and aspiration pneumonitis, wherein inhalation of gastric contents causes inflammation without the subsequent development of bacterial infection.[7, 8] Central to the study of aspiration pneumonia is whether it should exist as its own entity, or if aspiration is really a designation used for pneumonia in an older patient with greater comorbidities. The ability to clearly understand how a clinician diagnoses aspiration pneumonia, and whether that method has face validity with expert definitions may allow for improved future research, improved generalizability of current or past research, and possibly better clinical care.
Several validated mortality prediction models exist for community‐acquired pneumonia (CAP) using a variety of clinical predictors, but their performance in patients with aspiration pneumonia is less well characterized. Most studies validating pneumonia severity scoring systems excluded aspiration pneumonia from their study population.[9, 10, 11] Severity scoring systems for CAP may not accurately predict disease severity in patients with aspiration pneumonia. The CURB‐65[9] (confusion, uremia, respiratory rate, blood pressure, age 65 years) and the eCURB[12] scoring systems are poor predictors of mortality in patients with aspiration pneumonia, perhaps because they do not account for patient comorbidities.[13] The pneumonia severity index (PSI)[10] might predict mortality better than CURB‐65 in the aspiration population due to the inclusion of comorbidities.
Previous studies have demonstrated that patients with aspiration pneumonia are older and have greater disease severity and more comorbidities.[13, 14, 15] These single‐center studies also demonstrated greater mortality, more frequent admission to an intensive care unit (ICU), and longer hospital lengths of stay in patients with aspiration pneumonia. These studies identified aspiration pneumonia by the presence of a risk factor for aspiration[15] or by physician billing codes.[13] In practice, however, the bedside clinician diagnoses a patient as having aspiration pneumonia, but the logic is likely vague and inconsistent. Despite the potential for variability with individual judgment, an aggregate estimation from independent judgments may perform better than individual judgments.[16] Because there is no gold standard for defining aspiration pneumonia, all previous research has been limited to definitions created by investigators. This multicenter study seeks to determine what clinical characteristics lead physicians to diagnose a patient as having aspiration pneumonia, and whether or not the clinician‐derived diagnosis is distinct and clinically useful.
Our objectives were to: (1) identify covariates associated with bedside clinicians diagnosing a pneumonia patient as having aspiration pneumonia; (2) compare aspiration pneumonia and nonaspiration pneumonia in regard to disease severity, patient demographics, comorbidities, and clinical outcomes; and (3) measure the performance of the PSI in aspiration pneumonia versus nonaspiration pneumonia.
PATIENTS AND METHODS
Study Design and Setting
We performed a secondary analysis of the Community‐Acquired Pneumonia Organization (CAPO) database, which contains retrospectively collected data from 71 hospitals in 16 countries between June 2001 and December 2012. In each participating center, primary investigators selected nonconsecutive, adult hospitalized patients diagnosed with CAP. To decrease systematic selection biases, the selection of patients with CAP for enrollment in the trial was based on the date of hospital admission. Each investigator completed a case report form that was transferred via the internet to the CAPO study center at the University of Louisville (Louisville, KY). A sample of the data collection form is available at the study website (
Inclusion and Exclusion Criteria
Patients 18 years of age and satisfying criteria for CAP were included in this study. A diagnosis of CAP required a new pulmonary infiltrate at time of hospitalization, and at least 1 of the following: new or increased cough; leukocytosis; leukopenia, or left shift pattern on white blood cell count; and temperature >37.8C or <35.6 C. We excluded patients with pneumonia attributed to mycobacterial or fungal infection, and patients infected with human immunodeficiency virus, as we believed these types of pneumonia differ fundamentally from typical CAP.
Patient Variables
Patient variables included presence of aspiration pneumonia, laboratory data, comorbidities, and measures of disease severity, including the PSI. The clinician made a clinical diagnosis of the presence or absence of aspiration for each patient by marking a box on the case report form. Outcomes included in‐hospital mortality, hospital length of stay up to 14 days, and time to clinical stability up to 8 days. All variables were obtained directly from the case report form. In accordance with previously published definitions, we defined clinical stability as the day the following criteria were all met: improved clinical signs (improved cough and shortness of breath), lack of fever for >8 hours, improving leukocytosis (decreased at least 10% from the previous day), and tolerating oral intake.[17, 18]
Statistical Analysis
Baseline characteristics of patients with aspiration and nonaspiration CAP were compared using 2 or Fisher exact tests for categorical variables and the Mann‐Whitney U test for continuous variables.
To determine which patient variables were important in the physician diagnosis of aspiration pneumonia, we performed logistic regression with initial covariates comprising the demographic, comorbidity, and disease severity measurements listed in Table 1. We included interactions between cerebrovascular disease and age, nursing home status, and confusion to improve model fit. We centered all variables (including binary indicators) according to the method outlined by Kraemer and Blasey to improve interpretation of the main effects.[19]
Aspiration Pneumonia, N=451 | Nonaspiration Pneumonia, N=4,734 | P Value | |
---|---|---|---|
|
|||
Demographics | |||
Age, y | 79 (6587) | 69 (5380) | <0.001 |
% Male | 59% | 60% | 0.58 |
Nursing home residence | 25% | 5% | <0.001 |
Recent (30 days) antibiotic use | 21% | 16% | 0.017 |
Comorbidities | |||
Cerebrovascular disease | 35% | 14% | <0.001 |
Chronic obstructive pulmonary disease | 25% | 27% | 0.62 |
Congestive heart failure | 23% | 19% | 0.027 |
Diabetes | 18% | 18% | 0.85 |
Cancer | 12% | 10% | 0.12 |
Renal disease | 10% | 11% | 0.53 |
Liver disease | 6% | 5% | 0.29 |
Disease severity | |||
Pneumonia severity index | 123 (99153) | 92 (68117) | <0.001 |
Confusion | 49% | 12% | <0.001 |
PaO2 <60 mm Hg | 43% | 33% | <0.001 |
BUN >30 g/dL | 42% | 23% | <0.001 |
Multilobar pneumonia | 34% | 28% | 0.003 |
Pleural effusion | 25% | 21% | 0.07 |
Respiratory rate >30 breaths/minute | 21% | 20% | 0.95 |
pH <7.35 | 13% | 5% | <0.001 |
Hematocrit <30% | 11% | 6% | 0.001 |
Temperature >37.8C or <35.6C | 9% | 7% | 0.30 |
Systolic blood pressure <90 mm Hg | 8% | 9% | 0.003 |
Sodium <130 mEq/L | 8% | 6% | 0.08 |
Heart rate >125 beats/minute | 8% | 5% | 0.71 |
Glucose >250 mg/dL | 6% | 7% | 0.06 |
Cavitary lesion | 0% | 0% | 0.67 |
Clinical outcomes | |||
In‐hospital mortality | 23% | 9% | <0.001 |
Intensive care unit admission | 19% | 13% | 0.002 |
Hospital length of stay, d | 9 (515) | 7 (412) | <0.001 |
Time to clinical stability, d | 8 (48) | 4 (38) | <0.001 |
To determine if aspiration pneumonia had worse clinical outcomes compared to nonaspiration pneumonia, multiple methods were used. To compare the differences between the 2 groups with respect to time to clinical stability and length of hospital stay, we constructed Kaplan‐Meier survival curves and Cox proportional hazards regression models. The log‐rank test was used to determine statistical differences between the Kaplan‐Meier survival curves. To compare the impact of aspiration on mortality in patients with CAP, we conducted a propensity scorematched analysis. We chose propensity score matching over traditional logistic regression to balance variables among groups and to avoid the potential for overfit and multicollinearity. We considered a variable balanced after matching if its standardized difference was <10. All variables in the propensity scorematched analysis were balanced.
Although our dataset contained minimal missing data, we imputed any missing values to maintain the full study population in the creation of the propensity score. Missing data were imputed using the aregImpute function of the hmisc package of R (The R Foundation for Statistical Computing, Vienna, Austria).[20, 21] We built the propensity score model using a variable selection algorithm described by Bursac et al.[22] Our model included variables for region (United States/Canada, Europe, Asia/Africa or Latin America) and the variables listed in Table 1, with the exception of the PSI and the 4 clinical outcomes. Given that previous analyses accounting for clustering by physician did not substantially affect our results,[23] our model did not include physician‐level variables and did not account for the clustering effects of physicians. Using the propensity scores generated from this model, we matched a case of aspiration CAP with a case of nonaspiration CAP.[24] We then constructed a general linear model using the matched dataset to obtain the magnitude of effect of aspiration on mortality.
We used receiver operating characteristic curves to define the diagnostic accuracy of the pneumonia severity index for the prediction of mortality among patients with aspiration pneumonia and those with nonaspiration pneumonia. SAS version 9.3 (SAS Institute, Cary, NC) and R version 2.15.3 (The R Foundation for Statistical Computing) were used for all analyses. P values of 0.05 were considered statistically significant in all analyses.
RESULTS
Our initial query, after exclusion criteria, yielded a study population of 5185 patients (Figure 1). We compared 451 patients diagnosed with aspiration pneumonia to 4734 with CAP (Figure 1). Patient characteristics are summarized in Table 1. Patients with aspiration pneumonia were older, more likely to live in a nursing home, had greater disease severity, and were more likely to be admitted to an ICU. Patients with aspiration pneumonia had longer adjusted hospital lengths of stay and took more days to achieve clinical stability than patients with nonaspiration pneumonia (Figure 2). After adjusting for all variables in Table 1, the Cox proportional hazards models demonstrated that aspiration pneumonia was associated with ongoing hospitalization (hazard ratio [HR] for discharge: 0.77, 95% confidence interval [CI]: 0.65‐0.91, P=0.002) and clinical instability (HR for attaining clinical stability: 0.72, 95% CI: 0.61‐0.84, P<0.001). Patients with aspiration pneumonia presented with greater disease severity than those with nonaspiration pneumonia. Although there was no difference between groups in regard to temperature, respiratory rate, hyponatremia, or presence of pleural effusions or cavitary lesions, all other measured indices of disease severity were worse in patients with aspiration pneumonia. Patients with aspiration pneumonia were more likely to have cerebrovascular disease than those with nonaspiration pneumonia. Aspiration pneumonia patients also had increased prevalence of congestive heart failure. There was no appreciable difference between groups among other measured comorbidities.
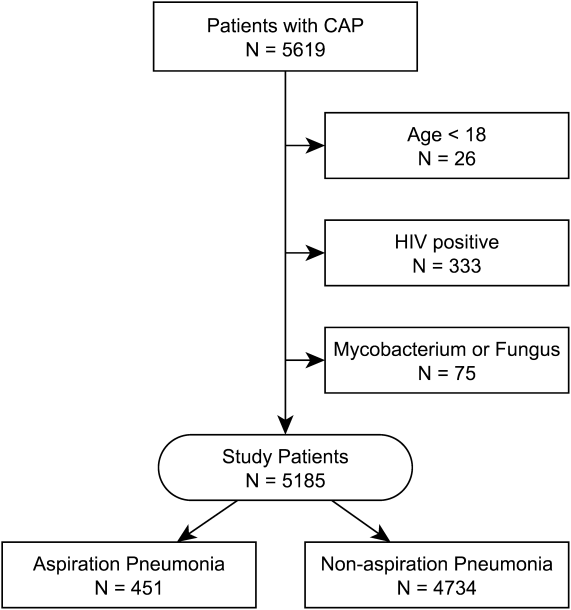
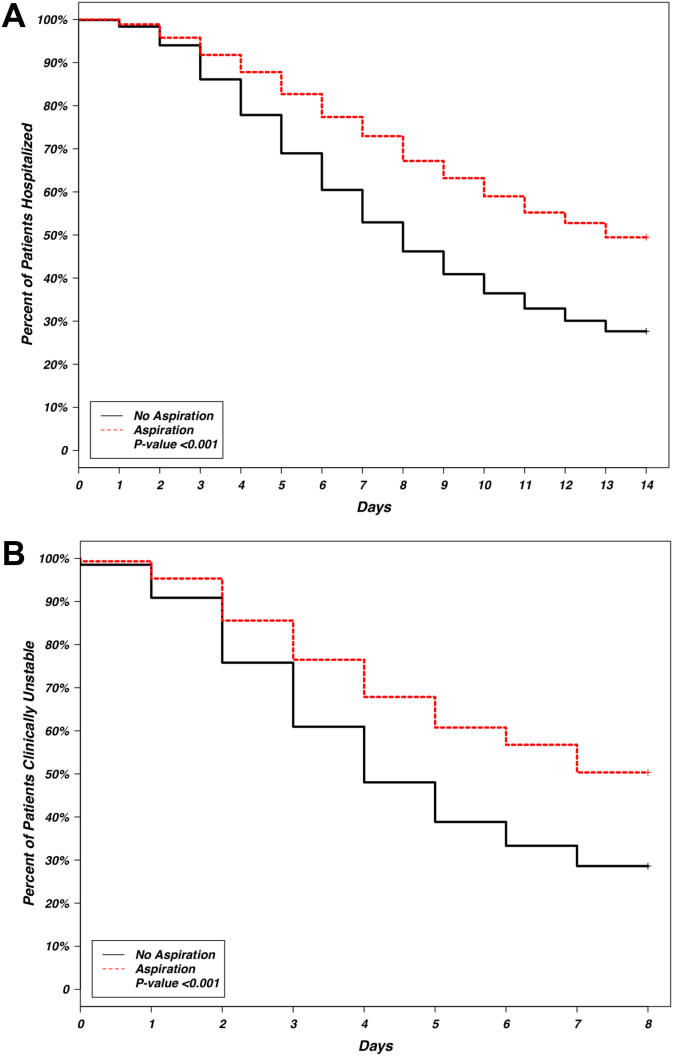
The patient characteristics most associated with a physician diagnosis of aspiration pneumonia, identified using logistic regression, were confusion, residence in nursing home, and presence of cerebrovascular disease (odds ratio [OR]: of 4.4, 2.9, and 2.3, respectively), whereas renal disease was associated with decreased physician diagnosis of aspiration pneumonia over nonaspiration pneumonia (OR: 0.58) (Table 2).
Covariate | Odds Ratio | 95% Confidence Intervals | P Value |
---|---|---|---|
|
|||
Demographics | |||
Age, y | 1.00 | 0.991.01 | 0.948 |
Male | 1.20 | 0.941.54 | 0.148 |
Nursing home residence | 2.93 | 2.134.00 | <0.001 |
Comorbidities | |||
Cerebrovascular disease | 2.26 | 1.533.32 | <0.001 |
Renal disease | 0.58 | 0.390.85 | 0.006 |
Disease severity | |||
Confusion | 4.41 | 3.405.72 | <0.001 |
Hematocrit <30% | 1.59 | 1.062.33 | 0.020 |
pH <7.35 | 1.67 | 1.102.47 | 0.013 |
Temperature >37.8C or <35.6C | 1.60 | 1.072.35 | 0.019 |
Multilobar pneumonia | 1.29 | 1.001.65 | 0.047 |
Interaction terms | |||
Age * cerebrovascular disease | 0.98 | 0.960.99 | 0.011 |
Nursing home * cerebrovascular disease | 0.51 | 0.270.96 | 0.037 |
Confusion * cerebrovascular disease | 0.70 | 0.421.17 | 0.175 |
Observed in‐patient mortality of aspiration pneumonia was 23%. This mortality was considerably higher than a mean PSI score of 123 would predict (class IV risk group, with expected 30‐day mortality of 8%9%[25]). The PSI score's ability to predict inpatient mortality in patients with aspiration pneumonia was moderate, with an area under the curve (AUC) of 0.71. This was similar to its performance in patients with nonaspiration pneumonia (AUC of 0.75) (Figure 3). These values are lower than the AUC of 0.81 for the PSI in predicting mortality derived from a meta‐analysis of 31 other studies.[26]
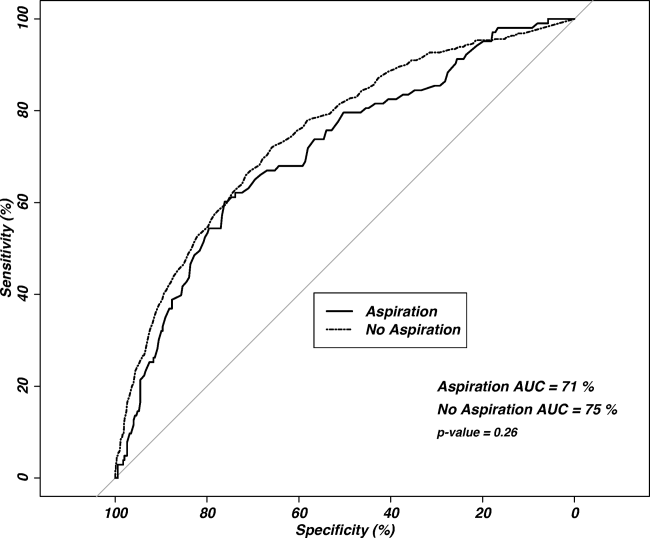
Our regression model after propensity score matching demonstrated that aspiration pneumonia independently confers a 2.3‐fold increased odds for inpatient mortality (95% CI: 1.56‐3.45, P<0.001).
DISCUSSION
Pneumonia patients with confusion, nursing home residence, or cerebrovascular disease are more likely to be diagnosed with aspiration pneumonia by clinicians. Although this is unsurprising, it is notable that these patients are more than twice as likely to die in the inpatient setting, even after accounting for age, comorbidities, and disease severity. These findings are similar to three previously published studies comparing aspiration and nonaspiration pneumonia at single institutions, albeit using different aspiration pneumonia definitions.[13, 14, 15] This study is the first large, multicenter, multinational study to demonstrate these findings.
Central to the interpretation of our results is the method of diagnosing aspiration versus nonaspiration. A bottom‐up method that relies on a clinician to check a box for aspiration may appear poorly reproducible. Because there is no diagnostic gold standard, clinicians may use different criteria to diagnose aspiration, creating potential for idiosyncratic noise. The strength of the wisdom of the crowd method used in this study is that an aggregate estimation from independent judgments may reduce the noise from individual judgments.[16] Although clinicians may vary in why they diagnose a particular patient as having aspiration pneumonia, it appears that the overwhelming reason for diagnosing a patient as having aspiration pneumonia is the presence of confusion, followed by previous nursing home residence or cerebrovascular disease. This finding has some face validity when compared with studies using an investigator definition, as altered mental status, chronic debility, and cerebrovascular disease are either prominent features of the definition of aspiration pneumonia[8] or frequently observed in patients with aspiration pneumonia.[13, 15] The distribution of cerebrovascular disease among our study's aspiration and nonaspiration pneumonia patients was similar to studies that used formal criteria in their definitions.[13, 15] Although nursing home residence was more likely in aspiration pneumonia patients, the majority of aspiration pneumonia patients were residing in the community, suggesting that aspiration is not simply a surrogate for healthcare‐associated pneumonia. Although patients with aspiration pneumonia are typically older than their nonaspiration counterparts, it appears that age is not a key determinant in the diagnosis of aspiration. With aspiration pneumonia, confusion, nursing home residence, and the presence of cerebrovascular disease are the greatest contributors in the clinical diagnosis, more than age.
Our data demonstrate that aspiration pneumonia confers increased odds for mortality, even after adjustment for age, disease severity, and comorbidities. These data suggest that aspiration pneumonia is a distinct entity from nonaspiration pneumonia, and that this disease is worse than nonaspiration CAP. If aspiration pneumonia is distinct from nonaspiration pneumonia, some unrecognized host factor other than age, disease severity, or the captured comorbidities decreases survival in aspiration pneumonia patients. However, it is also possible that aspiration pneumonia is merely a clinical designation for one end of the pneumonia spectrum, and we and others have failed to completely account for all measures of disease severity or all measures of comorbidities. Examples of unmeasured comorbidities would include presence of oropharyngeal dysphagia, which is not assessed in the database but could have a significant effect on clinical diagnosis. Unmeasured covariates can include measures beyond that of disease severity or comorbidity, such as the presence of a do not resuscitate (DNR) order, which could have a significant confounding effect on the observed association. A previous, single‐center study demonstrated that increased 30‐day mortality in aspiration pneumonia was mostly attributable to greater disease severity and comorbidities, although aspiration pneumonia independently conferred greater risk for adverse long‐term outcomes.[15] We propose that aspiration pneumonia represents a clinically distinct entity from nonaspiration pneumonia. Patients with chronic aspiration are often chronically malnourished and may have different oral flora than patients without chronic aspiration.[27, 28] Chronic aspiration has been associated with granulomatous reaction, organizing pneumonia, diffuse alveolar damage, and chronic bronchiolitis.[29] Chronic aspiration may elicit changes in the host physiology, and may render the host more susceptible to the development of secondary bacterial infection with morbid consequences.
The ability of the PSI to predict inpatient mortality was moderate (AUC only 0.7), with no significant additional discrimination between the aspiration and nonaspiration pneumonia groups. Although the PSI had moderate ability to predict inpatient mortality, the observed mortality was considerably higher than predicted. It is possible that the PSI incompletely captures clinically relevant comorbidities (eg, malnutrition). Further study to improve mortality prediction of aspiration pneumonia patients could employ sensitivity analysis to determine optimal thresholds and weighting of the PSI components.
Patients with aspiration pneumonia had longer hospital lengths of stay and took longer to achieve clinical stability than their nonaspiration counterparts. Time to clinical stability has been associated with increased posthospitalization mortality and is associated with time to switch from intravenous to oral antibiotics.[17] Although some component of hospital length‐of‐stay is subject to local practice patterns, time to clinical stability has explicit criteria for clinical improvement and failure, and therefore is less likely to be affected by local practice patterns.
We noted a relatively high (16%21%) incidence of prior antibiotic use among patients in this database. Analysis of antibiotic prescription patterns was limited, given the several different countries from which the database draws its cases. Although we used accepted criteria to define CAP cases, it is possible that this population may have a higher rate of resistant or uncommon pathogens than other studies of CAP that have populations with lower incidence of prior antibiotic use. Although not assessed, we suspect a significant component of the prior antibiotic use represented outpatient pneumonia treatment during the few days prior to visiting the hospital.
This study has several limitations, of which the most important may be that we used clinical determination for defining presence of aspiration pneumonia. This method is susceptible to the subjective perceptions of the treating clinician. We did not account for the effect of individual physicians in our model, although we did adjust for regional differences. The retrospective identification of patients allows for the possibility of selection bias, and therefore we have not attempted to make inferences regarding the relative incidence of pneumonia, nor did we adjust for temporal trends in diagnosis. The ratio of aspiration pneumonia patients to nonaspiration pneumonia patients may not necessarily reflect that observed in reality. Microbiologic and antibiotic data were unavailable for analysis. This study cannot inform on nonhospitalized patients with aspiration pneumonia, as only hospitalized patients were enrolled. The database identified cases of pneumonia, so it is possible for a patient to enter into the database more than once. Detection of mortality was limited to the inpatient setting rather than a set interval of 30 days. Inpatient mortality depends on length‐of‐stay patterns that may bias the mortality endpoint.[30] Also not assessed was the presence of a DNR order. It is possible that an older patient with greater comorbidities and disease severity may have care intentionally limited or withdrawn early by the family or clinicians.
Strengths of the study include its size and its multicenter, multinational population. The CAPO database is a large and well‐described population of patients with CAP.[17, 31] These attributes, as well as the clinician‐determined diagnosis, increase the generalizability of the study compared to a single‐center, single‐country study that employs investigator‐defined criteria.
CONCLUSION
Pneumonia patients with confusion, who are nursing home residence, and have cerebrovascular disease are more likely to be diagnosed with aspiration pneumonia by clinicians. Our clinician‐diagnosed cohort appears similar to those derived using an investigator definition. Patients with aspiration pneumonia are older, and have greater disease severity and more comorbidities than patients with nonaspiration pneumonia. They have greater mortality than their PSI score class would predict. Even after accounting for age, disease severity, and comorbidities, the presence of aspiration pneumonia independently conferred a greater than 2‐fold increase in inpatient mortality. These findings together suggest that aspiration pneumonia should be considered a distinct entity from typical pneumonia, and that additional research should be done in this field.
ACKNOWLEDGMENTS
Disclosures: M.J.L. contributed to the study design, data analysis, statistical analysis, and writing of the manuscript. P.P. contributed to the study design and revision of the manuscript for important intellectual content. T.W. and E.W. contributed to the study design, statistical analysis, and revision of the manuscript for important intellectual content. J.A.R. and N.C.D. contributed to the study design and revision of the manuscript for important intellectual content. All authors read and approved the final manuscript. M.L. takes responsibility for the integrity of the work as a whole, from inception to published article. This investigation was partly supported with funding from the National Center for Research Resources and the National Center for Advancing Translational Sciences, National Institutes of Health (grant 8UL1TR000105 [formerly UL1RR025764]). The authors report no conflicts of interest.
- Severe community‐acquired pneumonia. Epidemiology and prognostic factors. Am Rev Respir Dis. 1991;144(2):312–318. , , , et al.
- Risk factors for pneumonia in the elderly. Am J Med. 1994;96(4):313–320. , , .
- Aspiration pneumonia and dysphagia in the elderly. Chest. 2003;124(1):328–336. , .
- Pneumonia versus aspiration pneumonitis in nursing home residents: diagnosis and management. J Am Geriatr Soc. 2003;51(1):17–23. , , .
- Aspiration pneumonia: mixing apples with oranges and tangerines. Crit Care Med. 2004;32(5):1236; author reply 1236–1237. .
- Epidemiology and impact of aspiration pneumonia in patients undergoing surgery in Maryland, 1999–2000. Crit Care Med. 2003;31(7):1930–1937. , , , , .
- Aspiration syndromes: aspiration pneumonia and pneumonitis. Hosp Pract (Minneap). 2010;38(1):35–42. .
- Aspiration pneumonitis and aspiration pneumonia. N Engl J Med. 2001;344(9):665–671. .
- Defining community acquired pneumonia severity on presentation to hospital: an international derivation and validation study. Thorax. 2003;58(5):377–382. , , , et al.
- Comparison of a disease‐specific and a generic severity of illness measure for patients with community‐acquired pneumonia. J Gen Intern Med. 1995;10(7):359–368. , , , et al.
- Development and validation of a clinical prediction rule for severe community‐acquired pneumonia. Am J Respir Crit Care Med. 2006;174(11):1249–1256. , , , et al.
- CURB‐65 pneumonia severity assessment adapted for electronic decision support. Chest. 2011;140(1):156–163. , , , et al.
- Mortality, morbidity, and disease severity of patients with aspiration pneumonia. J Hosp Med. 2013;8(2):83–90. , , , .
- Pneumonia Severity Index (PSI), CURB‐65, and mortality in hospitalized elderly patients with aspiration pneumonia [in German]. Z Gerontol Geriatr. 2011;44(4):229–234. , , , , .
- Risk factors for aspiration in community‐acquired pneumonia: analysis of a hospitalized UK cohort. Am J Med. 2013;126(11):995–1001. , , , , .
- The wisdom of the crowd in combinatorial problems. Cogn Sci. 2012;36(3):452–470. , , , .
- Association between time to clinical stability and outcomes after discharge in hospitalized patients with community‐acquired pneumonia. Chest. 2011;140(2):482–488. , , , et al.
- Clinical stability and switch therapy in hospitalised patients with community‐acquired pneumonia: are we there yet? Eur Respir J. 2013;41(1):5–6. .
- Centring in regression analyses: a strategy to prevent errors in statistical inference. Int J Methods Psychiatr Res. 2004;13(3):141–151. , .
- http://CRAN.R‐project.org/package=Hmisc. Published Sept 12, 2014. Last accessed Oct 27, 2014. . Hmisc: Harrell miscellaneous. Available at:
- Multiple imputation for the fatal accident reporting system. J R Stat Soc Ser C Appl Stat. 1991;40(1):13–29. , .
- Purposeful selection of variables in logistic regression. Source Code Biol Med. 2008;3:17. , , , .
- CAPO authors. Mortality differences among hospitalized patients with community‐acquired pneumonia in three world regions: results from the Community‐Acquired Pneumonia Organization (CAPO) International Cohort Study. Respir Med. 2013;107(7):1101–1111. , , , , ;
- 2001:214–226. Available at: http://www2.sas.com/proceedings/sugi26/p214–26.pdf. Last accessed Oct 27, 2014. . Reducing bias in a propensity score matched‐pair sample using greedy matching techniques. In: Proceedings of the 26th Annual SAS Users Group International Conference. Cary, NC: SAS Institute Inc.;
- A prediction rule to identify low‐risk patients with community‐acquired pneumonia. N Engl J Med. 1997;336(4):243–250. , , , et al.
- Severity assessment tools for predicting mortality in hospitalised patients with community‐acquired pneumonia. Systematic review and meta‐analysis. Thorax. 2010;65(10):878–883. , , , et al.
- Prevalence and prognostic implications of dysphagia in elderly patients with pneumonia. Age Ageing. 2010;39(1):39–45. , , , , , .
- The association between oral microorgansims and aspiration pneumonia in the institutionalized elderly: review and recommendations. Dysphagia. 2010;25(4):307–322. , .
- Histopathology of aspiration pneumonia not associated with food or other particulate matter: a clinicopathologic study of 10 cases diagnosed on biopsy. Am J Surg Pathol. 2011;35(3):426–431. , .
- Interpreting hospital mortality data. The role of clinical risk adjustment. JAMA. 1988;260(24):3611–3616. , , , , , .
- Hospitalization for community‐acquired pneumonia: the pneumonia severity index vs clinical judgment. Chest. 2003;124(1):121–124. , , , .
- Severe community‐acquired pneumonia. Epidemiology and prognostic factors. Am Rev Respir Dis. 1991;144(2):312–318. , , , et al.
- Risk factors for pneumonia in the elderly. Am J Med. 1994;96(4):313–320. , , .
- Aspiration pneumonia and dysphagia in the elderly. Chest. 2003;124(1):328–336. , .
- Pneumonia versus aspiration pneumonitis in nursing home residents: diagnosis and management. J Am Geriatr Soc. 2003;51(1):17–23. , , .
- Aspiration pneumonia: mixing apples with oranges and tangerines. Crit Care Med. 2004;32(5):1236; author reply 1236–1237. .
- Epidemiology and impact of aspiration pneumonia in patients undergoing surgery in Maryland, 1999–2000. Crit Care Med. 2003;31(7):1930–1937. , , , , .
- Aspiration syndromes: aspiration pneumonia and pneumonitis. Hosp Pract (Minneap). 2010;38(1):35–42. .
- Aspiration pneumonitis and aspiration pneumonia. N Engl J Med. 2001;344(9):665–671. .
- Defining community acquired pneumonia severity on presentation to hospital: an international derivation and validation study. Thorax. 2003;58(5):377–382. , , , et al.
- Comparison of a disease‐specific and a generic severity of illness measure for patients with community‐acquired pneumonia. J Gen Intern Med. 1995;10(7):359–368. , , , et al.
- Development and validation of a clinical prediction rule for severe community‐acquired pneumonia. Am J Respir Crit Care Med. 2006;174(11):1249–1256. , , , et al.
- CURB‐65 pneumonia severity assessment adapted for electronic decision support. Chest. 2011;140(1):156–163. , , , et al.
- Mortality, morbidity, and disease severity of patients with aspiration pneumonia. J Hosp Med. 2013;8(2):83–90. , , , .
- Pneumonia Severity Index (PSI), CURB‐65, and mortality in hospitalized elderly patients with aspiration pneumonia [in German]. Z Gerontol Geriatr. 2011;44(4):229–234. , , , , .
- Risk factors for aspiration in community‐acquired pneumonia: analysis of a hospitalized UK cohort. Am J Med. 2013;126(11):995–1001. , , , , .
- The wisdom of the crowd in combinatorial problems. Cogn Sci. 2012;36(3):452–470. , , , .
- Association between time to clinical stability and outcomes after discharge in hospitalized patients with community‐acquired pneumonia. Chest. 2011;140(2):482–488. , , , et al.
- Clinical stability and switch therapy in hospitalised patients with community‐acquired pneumonia: are we there yet? Eur Respir J. 2013;41(1):5–6. .
- Centring in regression analyses: a strategy to prevent errors in statistical inference. Int J Methods Psychiatr Res. 2004;13(3):141–151. , .
- http://CRAN.R‐project.org/package=Hmisc. Published Sept 12, 2014. Last accessed Oct 27, 2014. . Hmisc: Harrell miscellaneous. Available at:
- Multiple imputation for the fatal accident reporting system. J R Stat Soc Ser C Appl Stat. 1991;40(1):13–29. , .
- Purposeful selection of variables in logistic regression. Source Code Biol Med. 2008;3:17. , , , .
- CAPO authors. Mortality differences among hospitalized patients with community‐acquired pneumonia in three world regions: results from the Community‐Acquired Pneumonia Organization (CAPO) International Cohort Study. Respir Med. 2013;107(7):1101–1111. , , , , ;
- 2001:214–226. Available at: http://www2.sas.com/proceedings/sugi26/p214–26.pdf. Last accessed Oct 27, 2014. . Reducing bias in a propensity score matched‐pair sample using greedy matching techniques. In: Proceedings of the 26th Annual SAS Users Group International Conference. Cary, NC: SAS Institute Inc.;
- A prediction rule to identify low‐risk patients with community‐acquired pneumonia. N Engl J Med. 1997;336(4):243–250. , , , et al.
- Severity assessment tools for predicting mortality in hospitalised patients with community‐acquired pneumonia. Systematic review and meta‐analysis. Thorax. 2010;65(10):878–883. , , , et al.
- Prevalence and prognostic implications of dysphagia in elderly patients with pneumonia. Age Ageing. 2010;39(1):39–45. , , , , , .
- The association between oral microorgansims and aspiration pneumonia in the institutionalized elderly: review and recommendations. Dysphagia. 2010;25(4):307–322. , .
- Histopathology of aspiration pneumonia not associated with food or other particulate matter: a clinicopathologic study of 10 cases diagnosed on biopsy. Am J Surg Pathol. 2011;35(3):426–431. , .
- Interpreting hospital mortality data. The role of clinical risk adjustment. JAMA. 1988;260(24):3611–3616. , , , , , .
- Hospitalization for community‐acquired pneumonia: the pneumonia severity index vs clinical judgment. Chest. 2003;124(1):121–124. , , , .
© 2014 Society of Hospital Medicine